ВУЗ: Казахская Национальная Академия Искусств им. Т. Жургенова
Категория: Учебное пособие
Дисциплина: Не указана
Добавлен: 03.02.2019
Просмотров: 17468
Скачиваний: 18
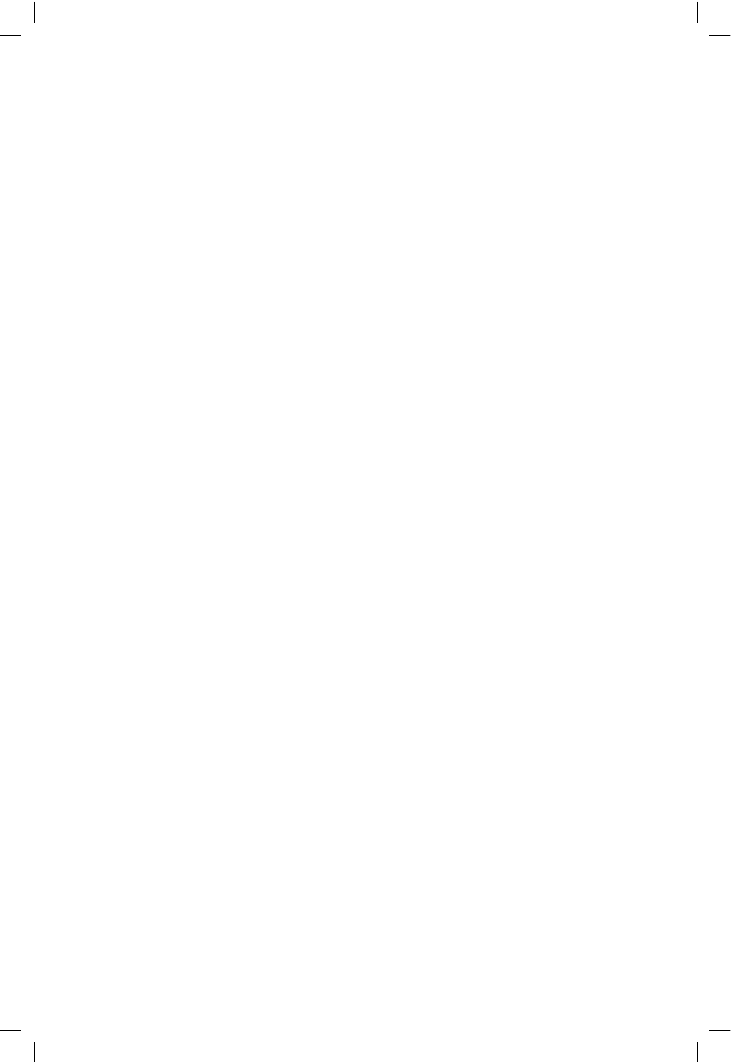
Grounding and practical matters
The internal space in the enclosure will require some ventilation to prevent
heat build-up; slots or small holes are desirable to keep foreign bodies out.
Avoid openings on the top surface as these will allow the entry of spilled
liquids, and increase dust entry. BS415 is a good starting point for this sort
of safety consideration, and this specifies that slots should be no more than
3 mm wide.
Reservoir electrolytics, unlike most capacitors, suffer significant internal
heating due to ripple current. Electrolytic capacitor life is very sensitive to
temperature, so mount them in the coolest position available, and if
possible leave room for air to circulate between them to minimise the
temperature rise.
Convection cooling
It is important to realise that the buoyancy forces that drive natural
convection are very small, and even small obstructions to flow can
seriously reduce the rate of flow, and hence the cooling. If ventilation is by
slots in the top and bottom of an amplifier case, then the air must be drawn
under the unit, and then execute a sharp right-angle turn to go up through
the bottom slots. This change of direction is a major impediment to air flow,
and if you are planning to lose a lot of heat then it feeds into the design of
something so humble as the feet the unit stands on; the higher the better, for
air flow. In one instance the amplifier feet were made 13 mm taller and all
the internal amplifier temperatures dropped by 5°C. Standing such a unit
on a thick-pile carpet can be a really bad idea, but someone is bound to do
it (and then drop their coat on top of it); hence the need for overtemperature
cutouts if amplifiers are to be fully protected.
Mains transformers
A toroidal transformer is useful because of its low external field. It must
be mounted so that it can be rotated to minimise the effect of what stray
fields it does emit. Most suitable toroids have single-strand secondary
lead-outs, which are too stiff to allow rotation; these can be cut short and
connected to suitably-large flexible wire such as 32/02, with carefully
sleeved and insulated joints. One prototype amplifier I have built had a
sizeable toroid mounted immediately adjacent to the TO3 end of the
amplifier PCB; however complete cancellation of magnetic hum (hum
and ripple output level below –90 dBu) was possible on rotation of the
transformer.
A more difficult problem is magnetic radiation caused by the reservoir
charging pulses (as opposed to the ordinary magnetisation of the core,
which would be essentially the same if the load current was sinusoidal)
which can be picked up by either the output connections or cabling to the
415
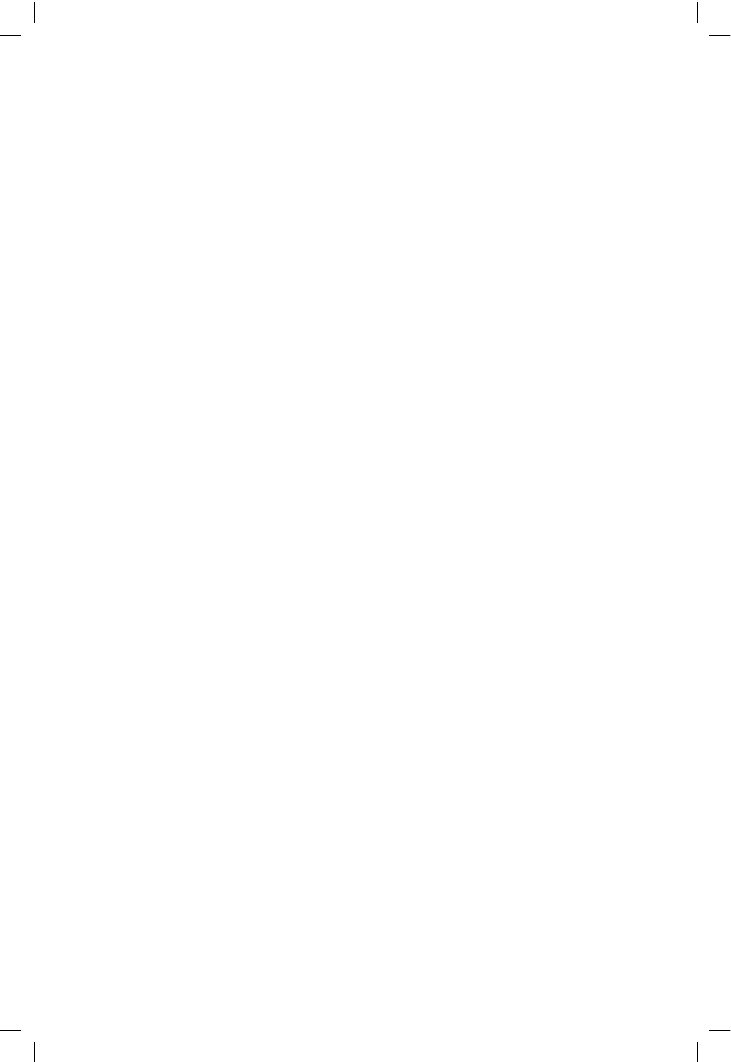
Audio Power Amplifier Design Handbook
power transistors if these are mounted off-board. For this reason the
transformer should be kept physically as far away as possible from even the
high-current section of the amplifier PCB.
As usual with toroids, ensure the bolt through the middle cannot form a
shorted turn by contacting the chassis in two places.
Wiring layout
There are several important points about the wiring for any power
amplifier:
!
Keep the + and – HT supply wires to the amplifiers close together. This
minimises the generation of distorted magnetic fields which may
otherwise couple into the signal wiring and degrade linearity. Sometimes
it seems more effective to include the 0 V line in this cable run; if so it
should be tightly braided to keep the wires in close proximity. For the
same reason, if the power transistors are mounted off the PCB, the
cabling to each device should be configured to minimise loop
formation.
!
The rectifier connections should go direct to the reservoir capacitor
terminals, and then away again to the amplifiers. Common impedance
in these connections superimposes charging pulses on the rail ripple
waveform, which may degrade amplifier PSRR.
!
Do not use the actual connection between the two reservoir capacitors
as any form of star point. It carries heavy capacitor-charging pulses that
generate a significant voltage drop even if thick wire is used. As Figure
14.1 shows, the star-point is tee-ed off from this connection. This is a
star-point only insofar as the amplifier ground connections split off from
here, so do not connect the input grounds to it, as distortion performance
will suffer.
Semiconductor installation
!
Driver transistor installation. These are usually mounted onto separate
heatsinks that are light enough to be soldered into the PCB without
further fixing. Silicone thermal washers ensure good thermal contact,
and spring clips are used to hold the package firmly against the sink.
Electrical isolation between device and heatsink is not normally
essential, as the PCB need not make any connection to the heatsink
fixing pads.
!
TO3P power transistor installation. These large flat plastic devices are
usually mounted on to the main heatsink with spring clips, which are not
only are rapid to install, but also generate less mechanical stress in the
package than bolting the device down by its mounting hole. They also
give a more uniform pressure onto the thermal washer material.
416
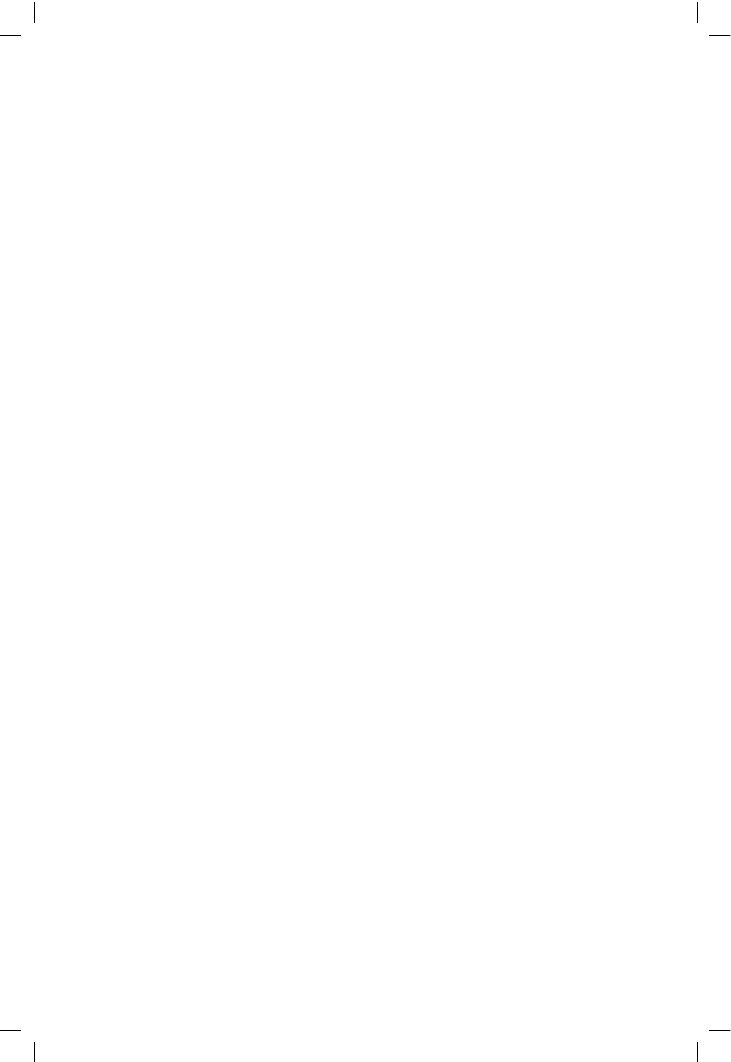
Grounding and practical matters
!
TO3 power transistor installation. The TO3 package is extremely
efficient at heat transfer, but notably more awkward to mount.
My preference is for TO3s to be mounted on an aluminium thermal-coupler
which is bolted against the component side of the PCB. The TO3 pins may
then be soldered directly on the PCB solder side. The thermal-coupler is
drilled with suitable holes to allow M3.5 fixing bolts to pass through the
TO3 flange holes, through the flange, and then be secured on the other side
of the PCB by nuts and crinkle washers which will ensure good contact
with the PCB mounting pads. For reliability the crinkle washers must cut
through the solder-tinning into the underlying copper; a solder contact
alone will creep under pressure and the contact force decay over time.
Insulating sleeves are essential around the fixing bolts where they pass
through the thermal-coupler; nylon is a good material for these as it has a
good high-temperature capability. Depending on the size of the holes
drilled in the thermal-coupler for the two TO3 package pins (and this
should be as small as practicable to maximise the area for heat transfer),
these are also likely to require insulation; silicone rubber sleeving carefully
cut to length is very suitable.
An insulating thermal washer must be used between TO3 and flange; these
tend to be delicate and the bolts must not be over-tightened. If you have a
torque-wrench, then 10 Newton/metre is an approximate upper limit for
M3.5 fixing bolts. Do not solder the two transistor pins to the PCB until the
TO3 is firmly and correctly mounted, fully bolted down, and checked for
electrical isolation from the heatsink. Soldering these pins and then
tightening the fixing bolts is likely to force the pads from the PCB. If this
should happen then it is quite in order to repair the relevant track or pad
with a small length of stranded wire to the pin; 7/02 size is suitable for a
very short run.
Alternatively, TO3s can be mounted off-PCB (e.g. if you already have a
large heatsink with TO3 drillings) with wires taken from the TO3 pads on
the PCB to the remote devices. These wires should be fastened together
(two bunches of three is fine) to prevent loop formation; see above. I cannot
give a maximum safe length for such cabling, but certainly 8 inches causes
no HF stability problems is my experience. The emitter and collector wires
should be substantial, e.g. 32/02, but the base connections can be as thin
as 7/02.
417
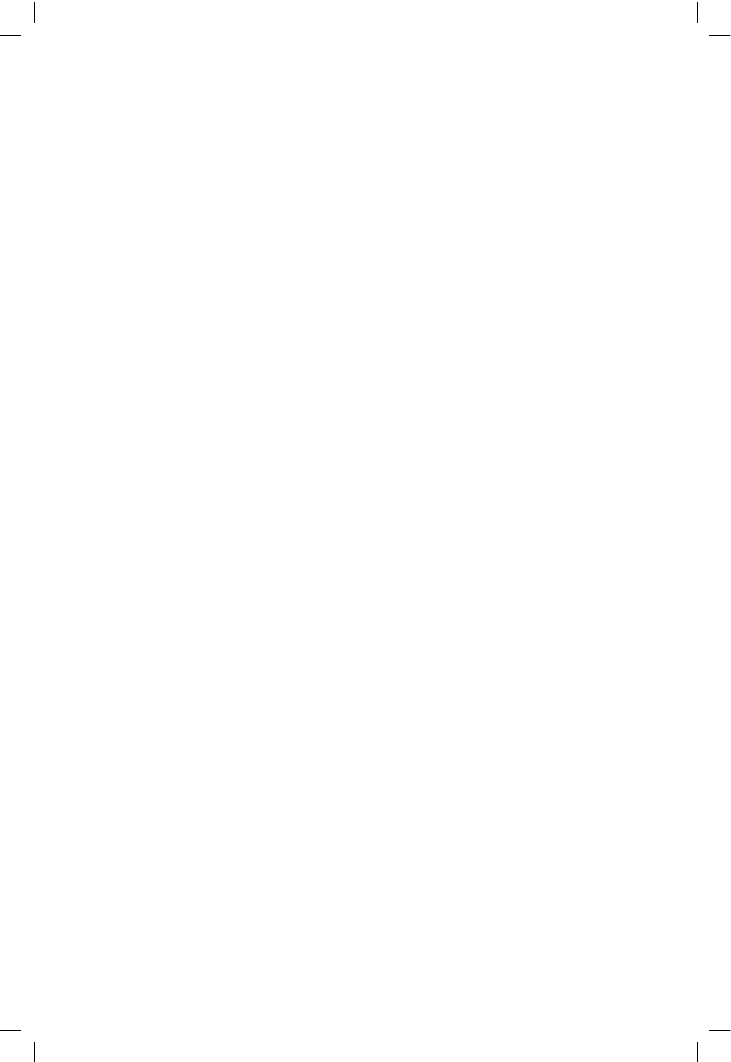
15
Testing and safety
Testing and fault-finding
Testing power amplifiers for correct operation is relatively easy; faultfinding
them when something is wrong is not. I have been professionally engaged
with power amplifiers for a long time, and I must admit I still sometimes
find it to be a difficult and frustrating business.
There are several reasons for this. Firstly, almost all small-signal audio
stages are IC-based, so the only part of the circuit likely to fail can be swiftly
replaced, so long as the IC is socketed. A power amplifier is the only place
where you are likely to encounter a large number of components all in one
big negative feedback loop. The failure of any components may (if you are
lucky) simply jam the amplifier output hard against one of the rails, or (if
you’re not) cause simultaneous failure of all the output devices, possibly
with a domino-theory trail of destruction winding through the small-signal
section. A certain make of high-power amplifier in the mid-70s was a
notorious example of the domino-effect, and when it failed (which was
often) the standard procedure was to replace all of the semiconductors,
back to and including the bridge rectifier. Component numbers here refer
to Figure 6.13.
By far the most important step to successful operation is a careful visual
inspection before switch-on. As in all power amplifier designs, a wrongly-
installed component may easily cause the immediate failure of several
others, making fault-finding difficult, and the whole experience generally
less than satisfactory. It is therefore most advisable to meticulously
check:
418
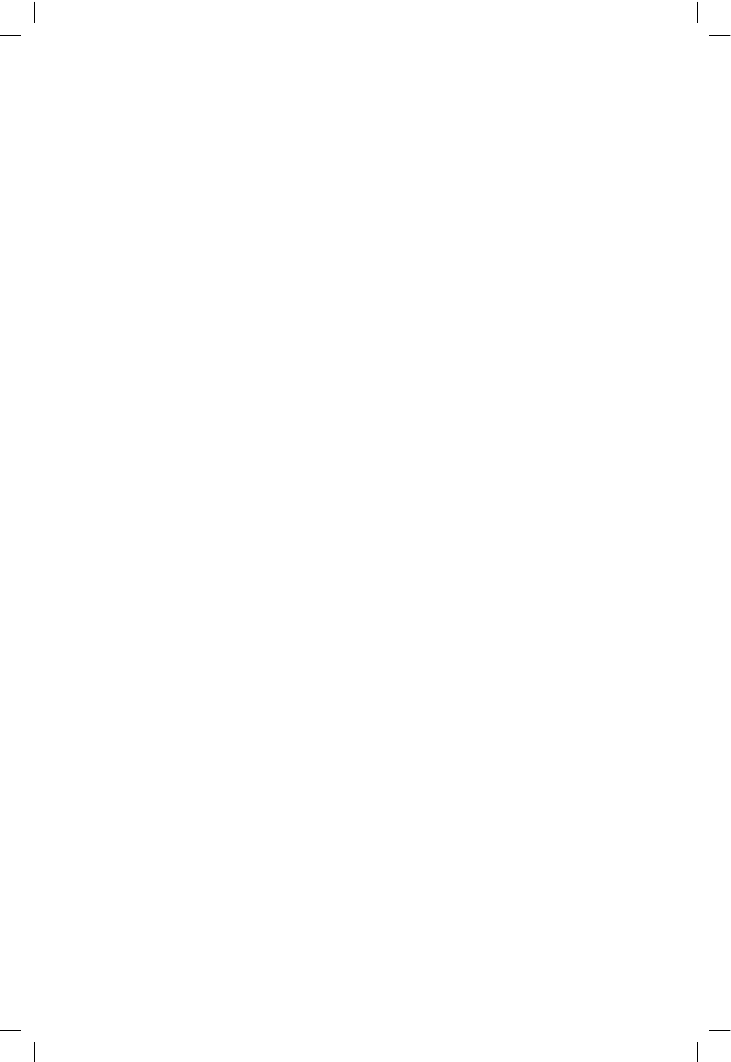
Testing and safety
!
That the supply and ground wiring is correct.
!
That all transistors are installed in the correct positions.
!
That the drivers and TO3 output devices are not shorted to their
respective heatsinks through faulty insulating washers.
!
That the circuitry around the bias generator TR13 in particular is
correctly built. An error here that leaves TR13 turned off will cause large
currents to flow through the output devices and may damage them
before the rail fuses can act.
For the Trimodal amplifier in Chapter 9, I recommend that the initial testing
is done in Class-B mode. There is the minimum amount of circuitry to
debug (the Class-A current-controller can be left disconnected, or not built
at all until later) and at the same time the Class-B bias generator can be
checked for its operation as a safety-circuit on Class-A/AB mode.
The second stage is to obtain a good sinewave output with no load
connected. A fault may cause the output to sit hard up against either
rail; this should not in itself cause any damage to components. Since a
power-amp consists of one big feedback loop, localising a problem can
be difficult. The best approach is to take a copy of the circuit diagram
and mark on it the DC voltage present at every major point. It should
then be straightforward to find the place where two voltages fail to
agree; e.g. a transistor installed backwards usually turns fully on, so the
feedback loop will try to correct the output voltage by removing all
drive from the base. The clash between full-on and no base-drive signals
the error.
When checking voltages in circuit, bear in mind that C2 is protected against
reverse voltage in both directions by diodes which will conduct if the
amplifier saturates in either direction.
This DC-based approach can fail if the amplifier is subject to high-
frequency oscillation, as this tends to cause apparently anomalous DC
voltages. In this situation the use of an oscilloscope is really essential. An
expensive oscilloscope is not necessary; a digital scope is at a disadvantage
here, because HF oscillation is likely to be aliased into nonsense and be
hard to interpret.
The third step is to obtain a good sinewave into a suitable high-wattage
load resistor. It is possible for faults to become evident under load that
are not shown up in Step 2 above.
Setting the quiescent conditions for any Class-B amplifier can only be
done accurately by using a distortion analyser. If you do not have access
to one, the best compromise is to set the quiescent voltage-drop across
both emitter resistors (R16, 17) to 10 mV when the amplifier is at
working temperature; disconnect the output load to prevent DC offsets
causing misleading current flow. This should be close to the correct
value, and the inherent distortion of this design is so low that minor
419