ВУЗ: Казахская Национальная Академия Искусств им. Т. Жургенова
Категория: Книга
Дисциплина: Не указана
Добавлен: 03.02.2019
Просмотров: 21671
Скачиваний: 19
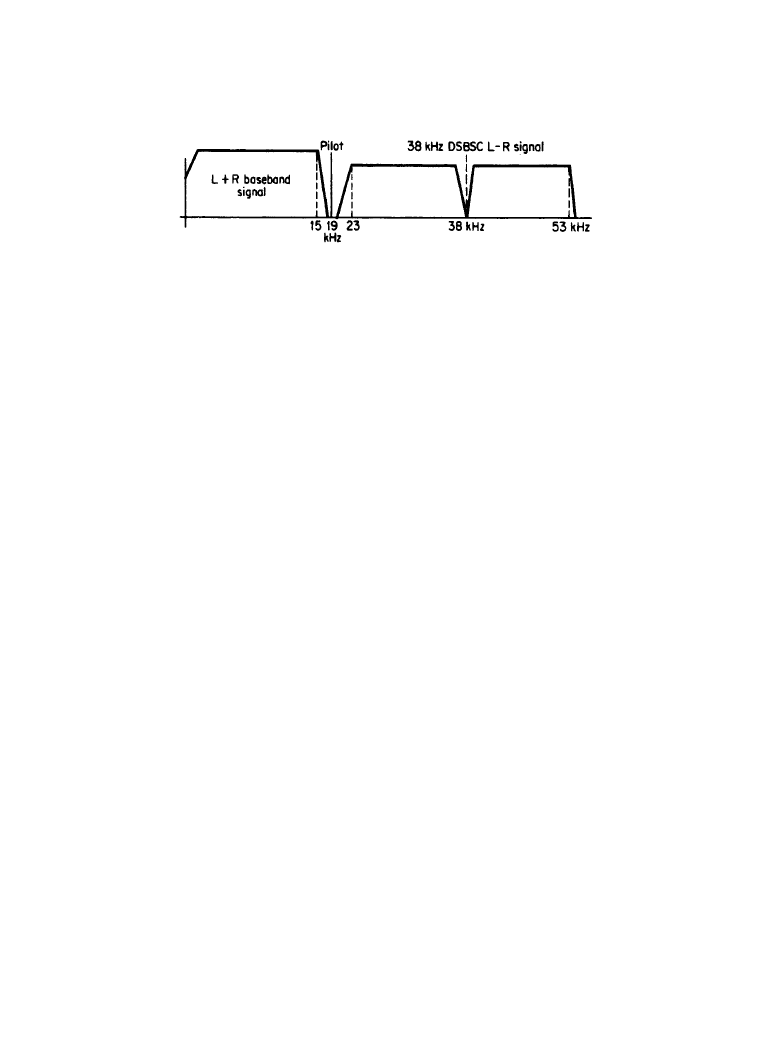
Radio Broadcasting 11-57
center frequency equal to the desired transmitter output frequency. In stereo broadcast systems, a
composite FM signal is applied to the FM modulator. The basic parameters of this composite
signal are shown in Figure 11.3.9.
Various techniques have been developed to generate the direct-FM signal. One of the most
popular uses a variable-capacity diode as the reactive element in the oscillator. The modulating
signal is applied to the diode, which causes the capacitance of the device to vary as a function of
the magnitude of the modulating signal. Variations in the capacitance cause the frequency of the
oscillator to vary. Again. the magnitude of the frequency shift is proportional to the amplitude of
the modulating signal, and the rate of frequency shift is equal to the frequency of the modulating
signal.
The direct-FM modulator is one element of an FM transmitter exciter, which generates the
composite FM waveform. A block diagram of a complete FM exciter is shown in Figure 11.3.10.
Audio inputs of various types (stereo left and right signals, plus subcarrier programming, if used)
are buffered, filtered, and preemphasized before being summed to feed the modulated oscillator.
It should be noted that the oscillator is not normally coupled directly to a crystal but is a free-run-
ning oscillator adjusted as near as practical to the carrier frequency of the transmitter. The final
operating frequency is carefully maintained by an automatic frequency control system employ-
ing a phase-locked loop (PLL) tied to a reference crystal oscillator or frequency synthesizer.
A solid-state class C amplifier follows the modulated oscillator and increases the operating
power of the FM signal to 20–30 W. One or more subsequent amplifiers in the transmitter raise
the signal power to several hundred watts for application to the final power amplifier (PA) stage.
Nearly all current high-power FM transmitters utilize solid-state amplifiers up to the final RF
stage, which is generally a vacuum tube for operating powers of 15 kW and above. All stages
operate in the class C mode.
11.3.3c
Subsidiary Communications Authorization (SCA)
In addition to its main channel and 38-kHz subcarrier stereo broadcasting, an FM station may
utilize additional subcarriers for private commercial use. These SCAs may be used in a variety of
ways, such as paging, data transmission, specialized foreign language programs, radio reading
services, utility load management, and background music. An FM stereo station may utilize mul-
tiplexed subcarriers within the range of 53–99 kHz with up to 20 percent total SCA modulation
of the main carrier using any form of modulation, including techniques for the transmission of
digital information. The only requirement is that the station does not exceed its occupied band-
width limitations or cause interference to itself.
Figure 11.3.9
Composite stereo FM signal.
Downloaded from Digital Engineering Library @ McGraw-Hill (www.digitalengineeringlibrary.com)
Copyright © 2004 The McGraw-Hill Companies. All rights reserved.
Any use is subject to the Terms of Use as given at the website.
Radio Broadcasting
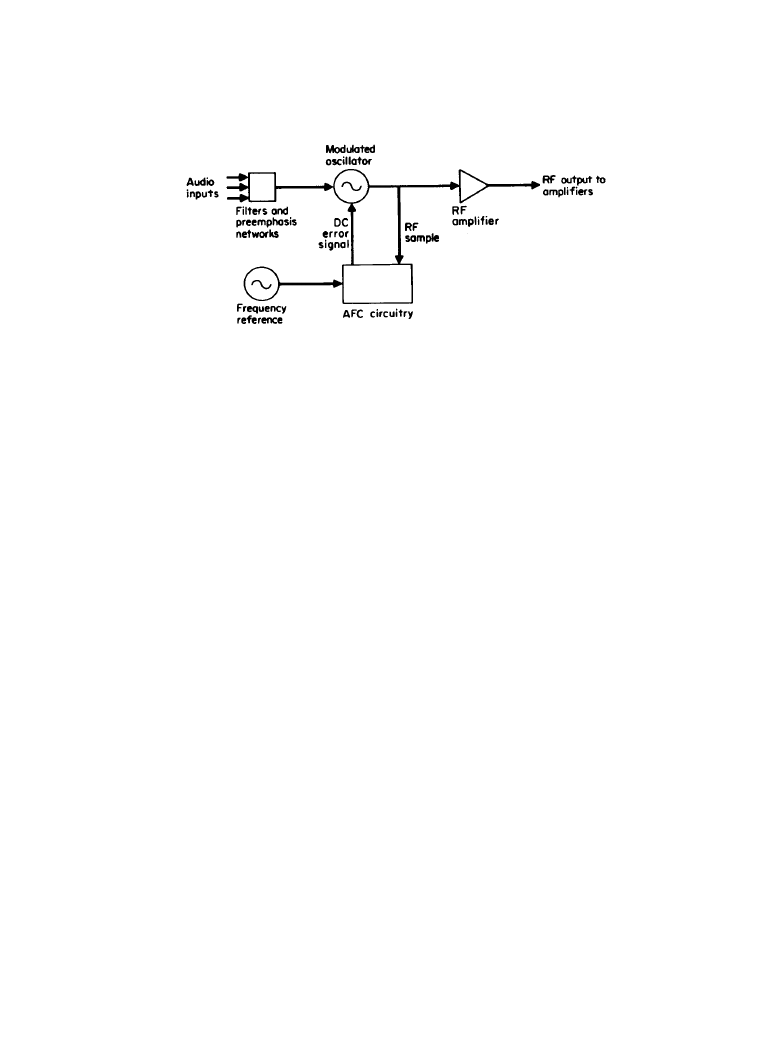
11-58 Broadcast Transmission Systems
A number of advanced modulation techniques have successfully been applied to transmission
of a high-speed digital data stream. These digital audio broadcasting (DAB) systems utilize in-
band on channel (IBOC) and in-band adjacent channel (IBAC) techniques to provide high-qual-
ity digital audio without interfering with the current analog FM broadcast signals.
11.3.3d
Frequency Allocations
The 100 carrier frequencies for FM broadcast range from 88.1 to 107.9 MHz and are equally
spaced every 200 kHz. The channels from 88.1 to 91.9 MHz are reserved for educational and
noncommercial broadcasting, and those from 92.1 to 107.9 MHz for commercial broadcasting.
Each channel has a 200-kHz bandwidth. The maximum frequency swing under normal condi-
tions is ±75 kHz. Stations operating with an SCA may, under certain conditions, exceed this
level, but in no case may exceed a frequency swing of ±82.5 kHz. The carrier center frequency is
required to be maintained within ±2000 Hz. The frequencies used for FM broadcasting limit the
coverage to essentially line-of-sight distances. As a result, consistent FM coverage is limited to a
maximum receivable range of a few hundred kilometers depending on the antenna height above
average terrain (HAAT) and effective radiated power (ERP). The actual coverage area for a given
station can be reliably predicted after the power and the antenna height are known. Either
increasing the power or raising the antenna will increase the coverage area.
In FM broadcast, stations are classified by the FCC according to their maximum allowable
ERP and the transmitting antenna HAAT in their service area. Class A stations provide primary
service to a radius of about 28 km with 6000 W of ERP at a maximum HAAT of 100 m. The
most powerful class, Class C, operates with maximums of 100,000 W of ERP and heights up to
600 m with a primary coverage radius of over 92 km. All classes may operate at antenna heights
above those specified but must reduce the ERP accordingly. Stations may not exceed the maxi-
mum power specified, even if the antenna height is reduced. The classification of the station
determines the allowable distance to other cochannel and adjacent channel stations.
Figure 11.3.10
Block diagram of an FM exciter.
Downloaded from Digital Engineering Library @ McGraw-Hill (www.digitalengineeringlibrary.com)
Copyright © 2004 The McGraw-Hill Companies. All rights reserved.
Any use is subject to the Terms of Use as given at the website.
Radio Broadcasting
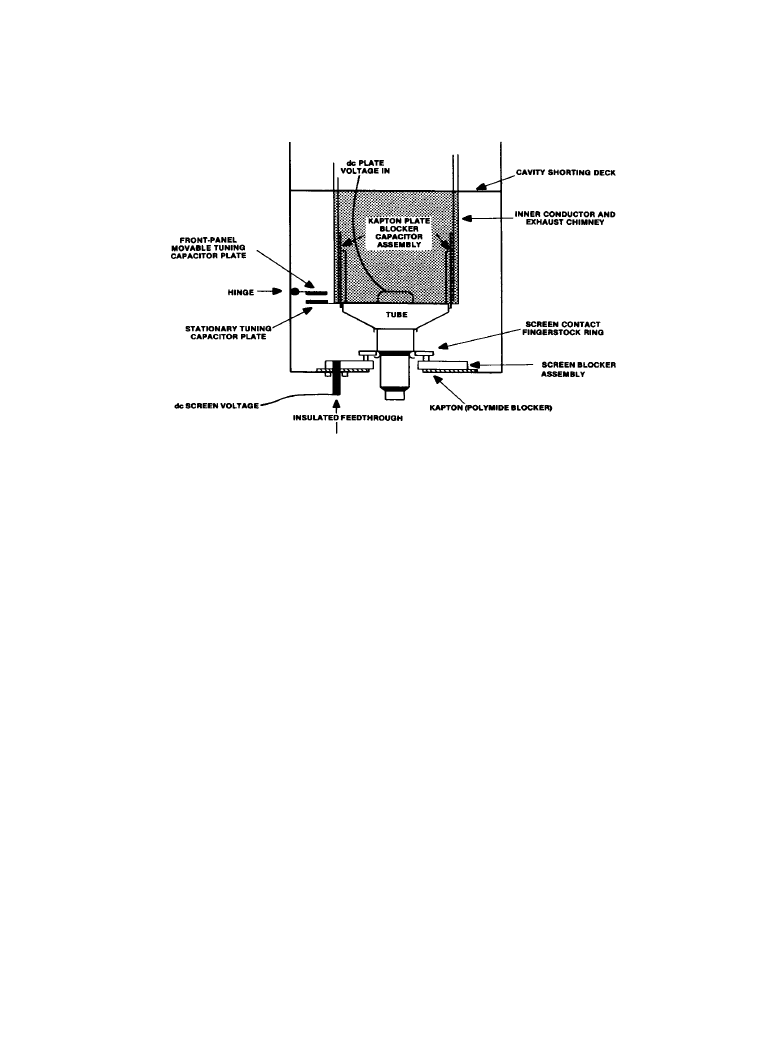
Radio Broadcasting 11-59
11.3.3e
Transmitters
FM broadcast transmitters typically range in power output from 10 W to 50 kW and have perfor-
mance standards similar to those specified above for AM broadcast transmitters. Digital technol-
ogy is being used extensively in the processing and exciter stages, allowing for precision
modulation control, whereas solid-state devices are predominately used for RF drivers and
amplifiers up to several kilowatts. High-power transmitters still rely on tubes in the final stages
for the generation of RF power, but manufacturers are developing new devices and technologies
that have made high-power solid-state transmitters cost effective and reliable.
FM Power Amplifiers
Nearly all high-power FM transmitters manufactured today employ cavity designs. The 1/4-
wavelength cavity is the most common. The design is simple and straightforward. A number of
variations can be found in different transmitters, but the underlying theory of operation is the
same.
The goal of any cavity amplifier is to simulate a resonant tank circuit at the operating fre-
quency and provide a means to couple the energy in the cavity to the transmission line. Because
of the operating frequencies involved (88 to 108 MHz), the elements of the “tank” take on unfa-
miliar forms.
A typical 1/4-wave cavity is shown in Figure 11.3.11. The plate of the tube connects directly
to the inner section (tube) of the plate-blocking capacitor. The blocking capacitor can be formed
in one of several ways. In at least one design, it is made by wrapping the outside surface of the
inner tube conductor with multiple layers of 8-in-wide and 0.005-in-thick polymide (Kapton)
film. The exhaust chimney-inner conductor forms the other element of the blocking capacitor.
The cavity walls form the outer conductor of the 1/4-wave transmission line circuit. The dc plate
Figure 11.3.11
Physical layout of a common type of 1/4-wave PA cavity for FM broadcast service.
Downloaded from Digital Engineering Library @ McGraw-Hill (www.digitalengineeringlibrary.com)
Copyright © 2004 The McGraw-Hill Companies. All rights reserved.
Any use is subject to the Terms of Use as given at the website.
Radio Broadcasting
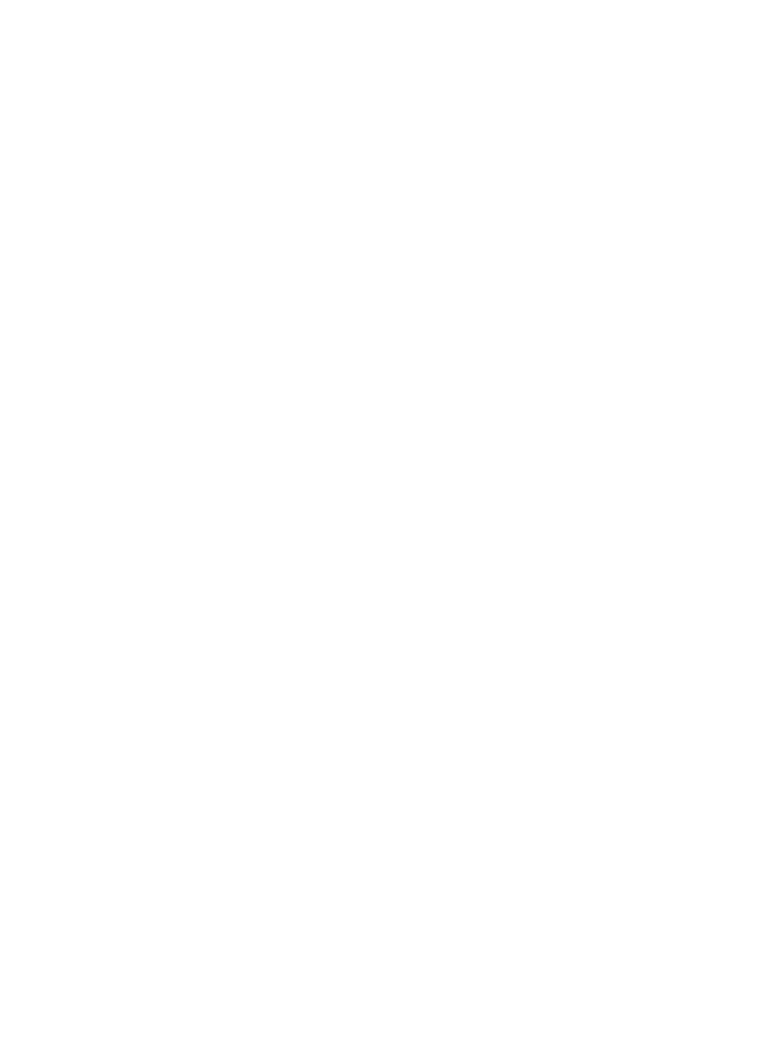
11-60 Broadcast Transmission Systems
voltage is applied to the PA tube by a cable routed inside the exhaust chimney and inner tube
conductor.
In the design shown in Figure 11.3.11, the screen-contact fingerstock ring mounts on a metal
plate that is insulated from the grounded-cavity deck by a Kapton blocker. This hardware makes
up the screen-blocker assembly. The dc screen voltage feeds to the fingerstock ring from under-
neath the cavity deck through an insulated feed through.
Some transmitters that employ the 1/4-wave cavity design use a grounded screen configura-
tion in which the screen contact fingerstock ring is connected directly to the grounded cavity
deck. The PA cathode then operates at below ground potential (in other words, at a negative volt-
age), establishing the required screen voltage for the tube.
The cavity design shown in Figure 11.3.11 is set up to be slightly shorter than a full 1/4-wave-
length at the operating frequency. This makes the load inductive and resonates the tube's output
capacity. Thus, the physically foreshortened shorted transmission line is resonated and electri-
cally lengthened to 1/4-wavelength.
Tuning the Cavity
Coarse tuning of the cavity is accomplished by adjusting the cavity length. The top of the cavity
(the cavity shorting deck) is fastened by screws or clamps and can be raised or lowered to set the
length of the assembly for the particular operating frequency.
Fine-tuning is accomplished by a variable-capacity plate-tuning control built into the cavity.
In the example, one plate of this capacitor, the stationary plate, is fastened to the inner conductor
just above the plate-blocking capacitor. The movable tuning plate is fastened to the cavity box,
the outer conductor, and is mechanically linked to the front-panel tuning control. This capacity
shunts the inner conductor to the outer conductor and can vary the electrical length and resonant
frequency of the cavity.
11.3.4 Transmitter Performance and Maintenance
Assessing the performance of an AM or FM transmitter has been greatly simplified by recent
advancements in RF system design. Most transmitters can be checked by measuring the audio
performance of the overall system. If the audio tests indicate good performance, chances are
good that all the RF parameters also meet specifications.
Both AM and FM broadcasting have certain limitations that prevent them from ever being a
completely transparent medium. Top on the list for FM is multipath distortion. In many loca-
tions, some degree of multipath is unavoidable. Running a close second is the practical audio
bandwidth limitation of the FM stereo multiplex system. The theoretical limit is 19 kHz; how-
ever, real-world filter designs result in a high-end passband of between 15 and 17 kHz.
For AM radio, the primary limitation is restricted bandwidth. Modern transmitters are capable
of faithfully transmitting wideband audio, but most receivers are not capable of reproducing it.
The problem is not just poor-quality AM radios but rather the high-noise environment of the AM
band. To reduce adjacent channel interference and atmospheric and artificial noise, receiver
designers have restricted the IF and audio bandwidth of their products. The result is a typical AM
receiver in which the audio response falls off rapidly beyond 3 kHz.
Downloaded from Digital Engineering Library @ McGraw-Hill (www.digitalengineeringlibrary.com)
Copyright © 2004 The McGraw-Hill Companies. All rights reserved.
Any use is subject to the Terms of Use as given at the website.
Radio Broadcasting
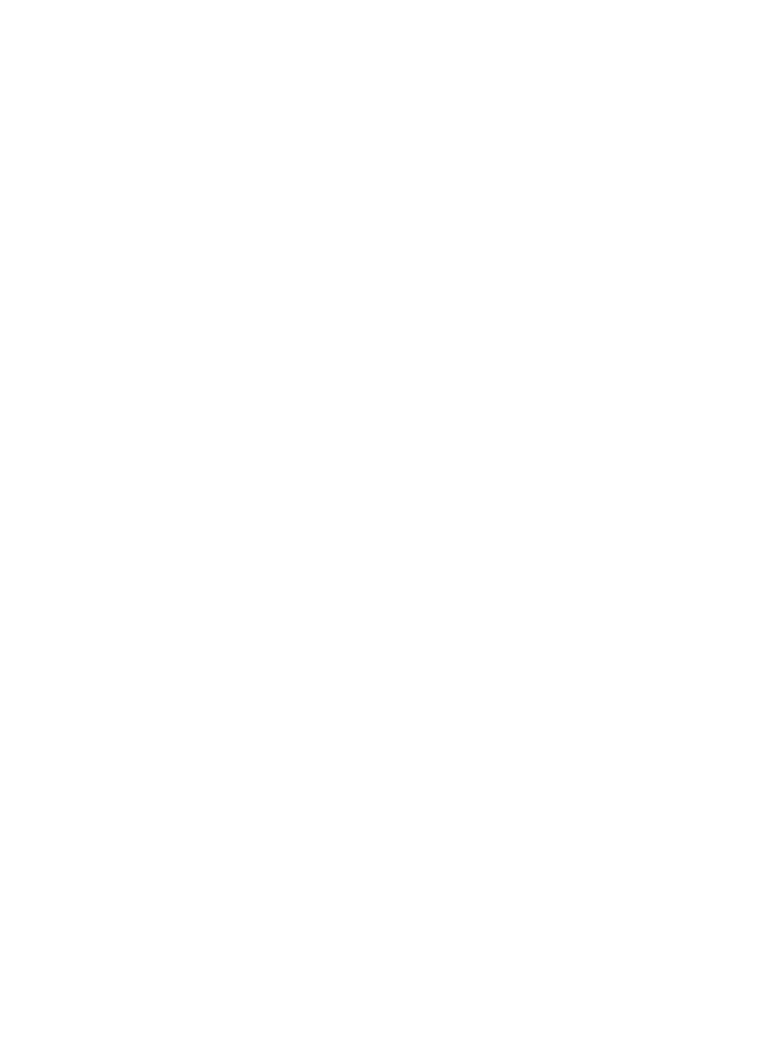
Radio Broadcasting 11-61
11.3.4a
Key System Measurements
The procedures for measuring AM and FNI transmitter performance vary widely depending on
the type of equipment being used. Some generalizations, however, can be made with respect to
equipment performance measurements that apply to most systems.
Frequency response is the actual deviation from a constant amplitude across a given span of
frequencies. Research has shown that frequency-response accuracy over the audio passband does
make an audible difference. Researchers exploring subtle differences in audio amplifier design
have found that errors as small as 0.2 dB can be heard. Therefore, fiat response (strict adherence
to the 75-
µs preemphasis curve) is an important goal in FM broadcasting. Typical performance
targets for an FM station are ±1 dB, 50 Hz to 15 kHz. Typical targets for an AM station are ±1
dB, 50 Hz to 10 kHz.
Total harmonic distortion (THD) is the creation by a nonlinear device of spurious signals har-
monically related to the applied audio waveform. Research has shown that although THD levels
greater than 1 percent are detectable during sinewave testing, listeners will tolerate somewhat
higher levels of THD on musical material. It must be noted that depending upon the setup of the
station's audio processor, distortion targets of 1 percent or less may be impossible to meet with
the processor on-line. Because of the highly competitive nature of broadcasting today, audio pro-
cessors cannot always be adjusted to provide for the purest reproduction of the incoming signal.
Audio processing aside, most FM transmitters will yield THD readings well below 1 percent
at frequencies up to 7.5 kHz. AM transmitters can typically produce distortion figures below 1.5
percent at frequencies up to 5 kHz at 95 percent modulation.
The THD test is sensitive to the noise floor of the system under test. If the system has a sig-
nal-to-noise ratio of 60 dB, the distortion analyzer's best possible reading will be greater than 0.1
percent (60 dB = 0.001 = 0.1 percent).
Intermodulation distortion (IMD) is the creation by a nonlinear device of spurious signals not
harmonically related to the audio waveform. These distortion components are sum-and-differ-
ence (beat notes) mixing products that research has shown are more objectionable to listeners
than even-harmonic distortion products. The IMD measurement is relatively impervious to the
noise floor of the system under test. IMD performance targets for AM and FM transmitters are
the same as the TH D targets mentioned previously.
Signal-to-noise ratio (S/N) is the amplitude difference, expressed in decibels, between a refer-
ence level audio signal and the system's residual noise and hum. In many cases, system noise is
the most difficult parameter to bring under control. An FM performance target of –70 dB per ste-
reo channel reflects state-of-the-art, exciter-transmitter performance. Most AM transmitters are
capable of –60 dB S/N for monaural operation, and –55 dB per channel for stereo operation.
Separation is a specialized definition for signal crosstalk between the left and right channels
of a stereo system. The separation test is performed by feeding a test lone into one channel while
measuring leakage into the other channel (whose input is terminated with a 600-
Ω wirewound
resistor, or other appropriate value). Typical performance targets for an FM station are –35 dB
from 50 to 400 Hz, and –40 dB from 400 Hz to 15 kHz. Targets for a stereo AM station are –20
dB from 50 to 40 Hz and –25 dB from 400 Hz to 10 kHz.
Synchronous AM in FM Systems
The output spectrum of an FM transmitter contains many sideband frequency components, theo-
retically an infinite number. The practical considerations of transmitter design and frequency
Downloaded from Digital Engineering Library @ McGraw-Hill (www.digitalengineeringlibrary.com)
Copyright © 2004 The McGraw-Hill Companies. All rights reserved.
Any use is subject to the Terms of Use as given at the website.
Radio Broadcasting