ВУЗ: Казахская Национальная Академия Искусств им. Т. Жургенова
Категория: Книга
Дисциплина: Не указана
Добавлен: 03.02.2019
Просмотров: 21649
Скачиваний: 19
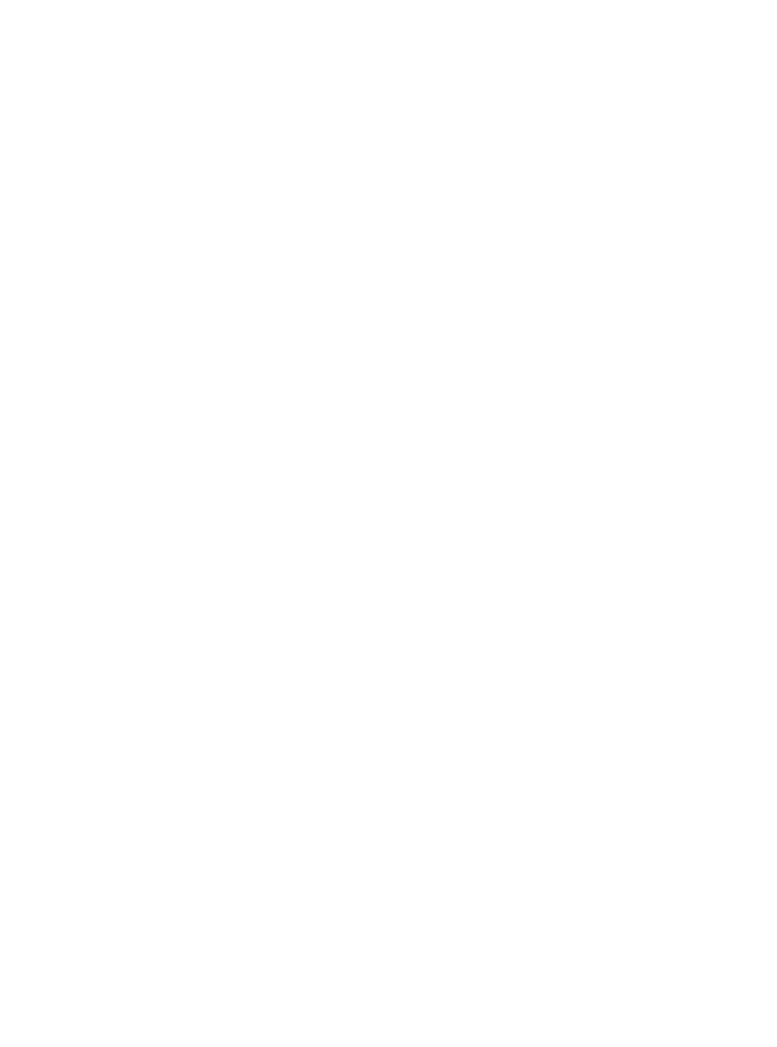
Transmitting Antennas 11-107
•
Radiating near-field region. This region is characterized by the predictable distribution of the
radiating field. In the near-field region, the relative angular distribution of the field is depen-
dent on the distance from the antenna.
•
Far-field region. This region is characterized by the independence of the relative angular dis-
tribution of the field with varying distance. The pattern is essentially independent of distance.
11.5.2f
Impedance Matching
Most practical antennas require some form of impedance matching between the transmission
line and the radiating elements. The implementation of a matching network can take on many
forms, depending upon the operating frequency and output power.
The negative sign convention is generally used in impedance matching analysis. That is, if a
network delays or retards a signal by
θ degrees, the phase shift across the network is said to be
minus
θ degrees. For example, a 1/4-wavelength of transmission line, if properly terminated, has
a phase shift of –90°. Thus a lagging or low-pass network has a negative phase shift, and a lead-
ing or high-pass network has a positive phase shift. There are three basic network types that can
be used for impedance matching: L, pi, and tee.
L Network
The L network is shown in Figure 11.5.2. The loaded Q of the network is determined from Equa-
tion (1). Equation (2) defines the shunt leg reactance, which is negative (capacitive) when
θ is
negative, and positive (inductive) when
θ is positive. The series leg reactance is found using
Equation (3), the phase shift via Equation (4), and the currents and voltages via Ohm's Law. Note
that R
2
(the resistance on the shunt leg side of the L network) must always be greater than R
1
. An
L network cannot be used to match equal resistances, or to adjust phase independently of resis-
tance.
Tee Network
The tee network is shown in Figure 11.5.3. This configuration can be used to match unequal
resistances. The tee network has the added feature that phase shift is independent of the resis-
tance transformation ratio. A tee network can be considered simply as two L networks back-to-
back. Note that there are two loaded Qs associated with a tee network: an input Q and output Q.
In order to gauge the bandwidth of the tee network, the lower value of Q must be ignored. Note
that the Q of a tee network increases with increasing phase shift.
Equations (5) through (14) describe the tee network. It is a simple matter to find the input and
output currents via Ohm's Law, and the shunt leg current can be found via the Cosine Law, Equa-
tion (12). Note that this current increases with increasing phase shift. Equation (13) describes the
mid-point resistance of a tee network, which is always higher than R
1
or R
2
. Equation (14) is use-
ful when designing a phantom tee network; that is, where X
2
is made up only of the antenna reac-
tance, and there is no physical component in place of X
2
. Keep in mind that a tee network is
considered as having a lagging or negative phase shift when the shunt leg is capacitive (X
3
nega-
tive), and vice versa. The input and output arms can go either negative or positive, depending on
the resistance transformation ratio and desired phase shift.
Downloaded from Digital Engineering Library @ McGraw-Hill (www.digitalengineeringlibrary.com)
Copyright © 2004 The McGraw-Hill Companies. All rights reserved.
Any use is subject to the Terms of Use as given at the website.
Transmitting Antennas
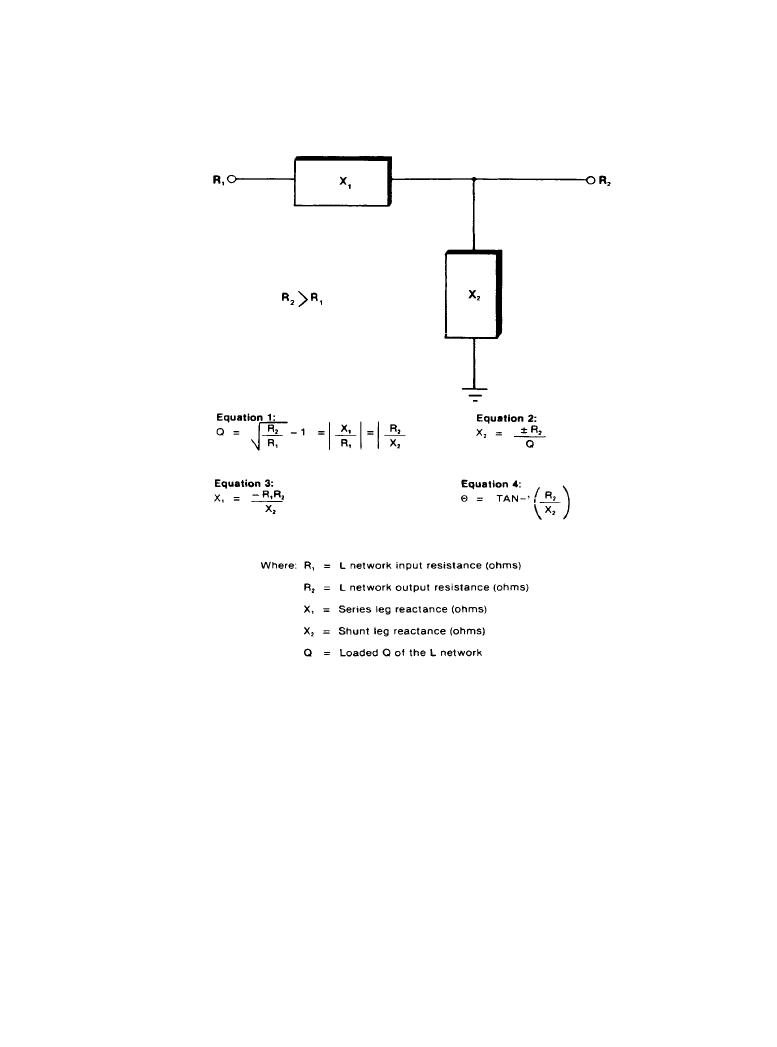
11-108 Broadcast Transmission Systems
Pi Network
The pi network is shown in Figure 11.5.4. It can also be considered as two L networks back-to-
back and, therefore, the same comments regarding overall loaded Q apply. Note that susceptan-
ces have been used in Equations (15) through (19) instead of reactances in order to simplify cal-
culations. The same conventions regarding tee network currents apply to pi network voltages,
Equations (20, 21, and 22). The mid-point resistance of a pi network is always less than R
1
or R
2
.
A pi network is considered as having a negative or lagging phase shift when Y
3
is positive, and
vice versa.
Figure 11.5.2
L network parameters.
Downloaded from Digital Engineering Library @ McGraw-Hill (www.digitalengineeringlibrary.com)
Copyright © 2004 The McGraw-Hill Companies. All rights reserved.
Any use is subject to the Terms of Use as given at the website.
Transmitting Antennas
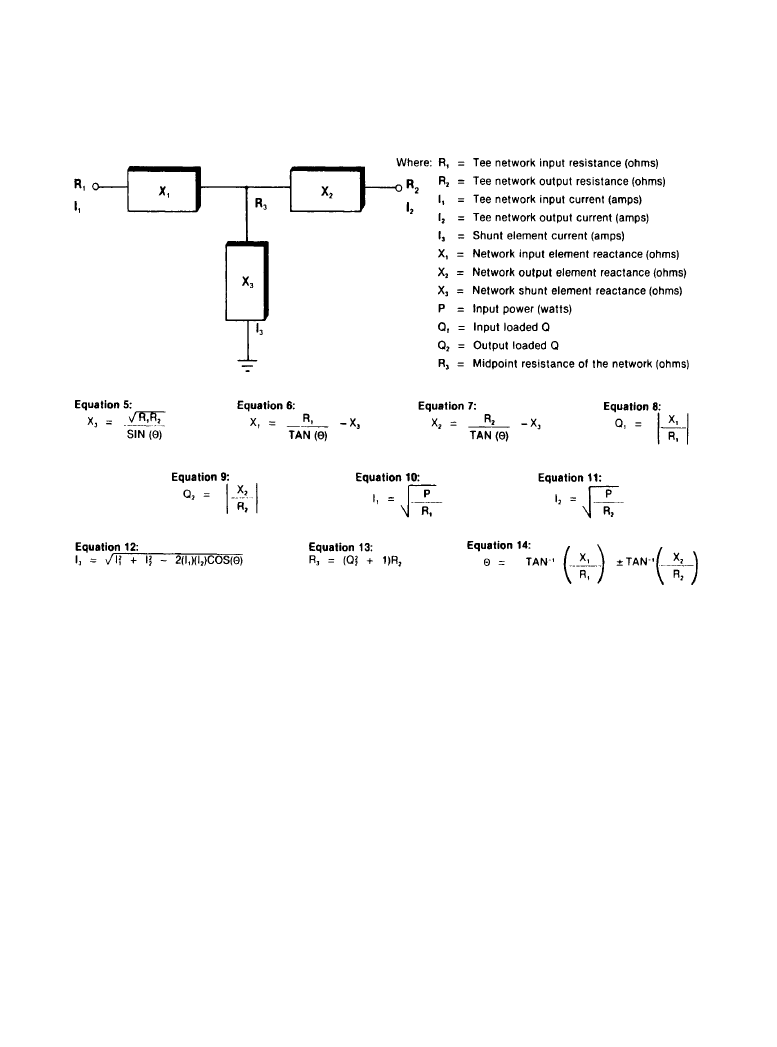
Transmitting Antennas 11-109
Line Stretcher
A line stretcher makes a transmission line look longer or shorter in order to produce sideband
impedance symmetry at the transmitter PA (see Figure 11.5.5). This is done to reduce audio dis-
tortion in an envelope detector—the kind of detector that most monophonic AM receivers
employ. Symmetry is defined as equal sideband resistances, and equal—but opposite sign—side-
band reactances.
There are two possible points of symmetry, each 90° from the other. One produces sideband
resistances greater than the carrier resistance, and the other produces the opposite effect. One
side will create a pre-emphasis effect, and the other a de-emphasis effect.
Depending on the Q of the transmitter output network, one point of symmetry may yield
lower sideband VSWR at the PA than the other. This results from the Q of the output network
opposing the Q of the antenna in one direction, but aiding the antenna Q in the other direction.
Figure 11.5.3
T network parameters.
Downloaded from Digital Engineering Library @ McGraw-Hill (www.digitalengineeringlibrary.com)
Copyright © 2004 The McGraw-Hill Companies. All rights reserved.
Any use is subject to the Terms of Use as given at the website.
Transmitting Antennas
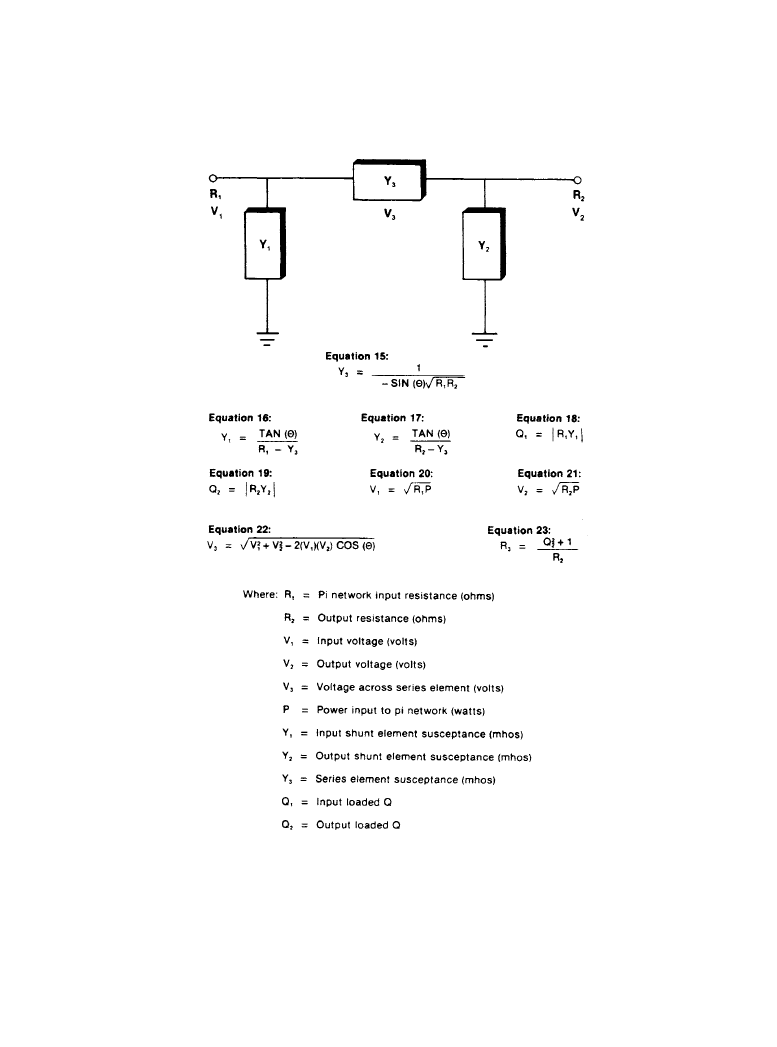
11-110 Broadcast Transmission Systems
Figure 11.5.4
Pi network parameters.
Downloaded from Digital Engineering Library @ McGraw-Hill (www.digitalengineeringlibrary.com)
Copyright © 2004 The McGraw-Hill Companies. All rights reserved.
Any use is subject to the Terms of Use as given at the website.
Transmitting Antennas
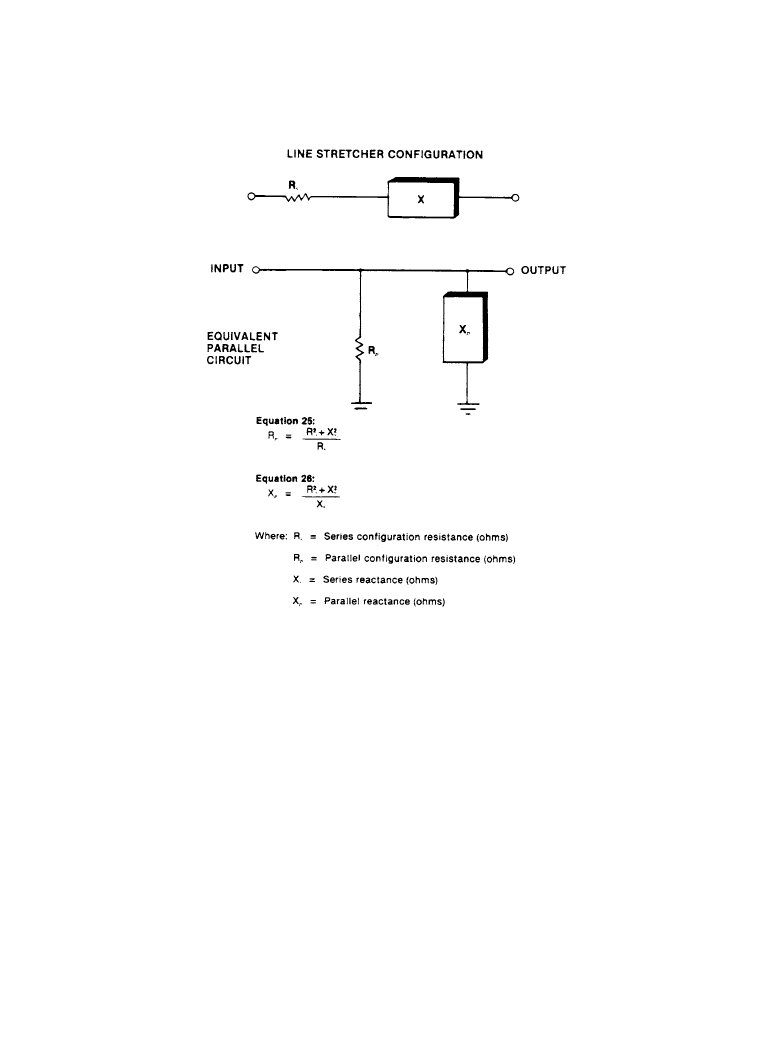
Transmitting Antennas 11-111
11.5.3 Antenna Types
The dipole antenna is simplest of all antennas, and the building block of most other designs. The
dipole consists of two in-line rods or wires with a total length equal to 1/2-wave at the operating
frequency. Figure 11.5.6 shows the typical configuration, with two 1/4-wave elements connected
to a transmission line. The radiation resistance of a dipole is on the order of 73
Ω. The bandwidth
of the antenna can be increased by increasing the diameter of the elements, or by using cones or
cylinders rather than wires or rods, as shown in the figure. Such modifications also increase the
impedance of the antenna.
The dipole can be straight (in-line) or bent into a V-shape. The impedance of the V-dipole is a
function of the V angle. Changing the angle effectively tunes the antenna. The vertical radiation
pattern of the V-dipole antenna is similar to the straight dipole for angles of 120° or less.
A folded dipole may be fashioned as shown in Figure 11.5.7. Such a configuration results in
increased bandwidth and impedance. Impedance can be further increased by using rods of differ-
ent diameter and by varying the spacing of the elements. The 1/4-wave dipole elements con-
Figure 11.5.5
Line stretcher configuration.
Downloaded from Digital Engineering Library @ McGraw-Hill (www.digitalengineeringlibrary.com)
Copyright © 2004 The McGraw-Hill Companies. All rights reserved.
Any use is subject to the Terms of Use as given at the website.
Transmitting Antennas