ВУЗ: Казахская Национальная Академия Искусств им. Т. Жургенова
Категория: Книга
Дисциплина: Не указана
Добавлен: 03.02.2019
Просмотров: 21628
Скачиваний: 19
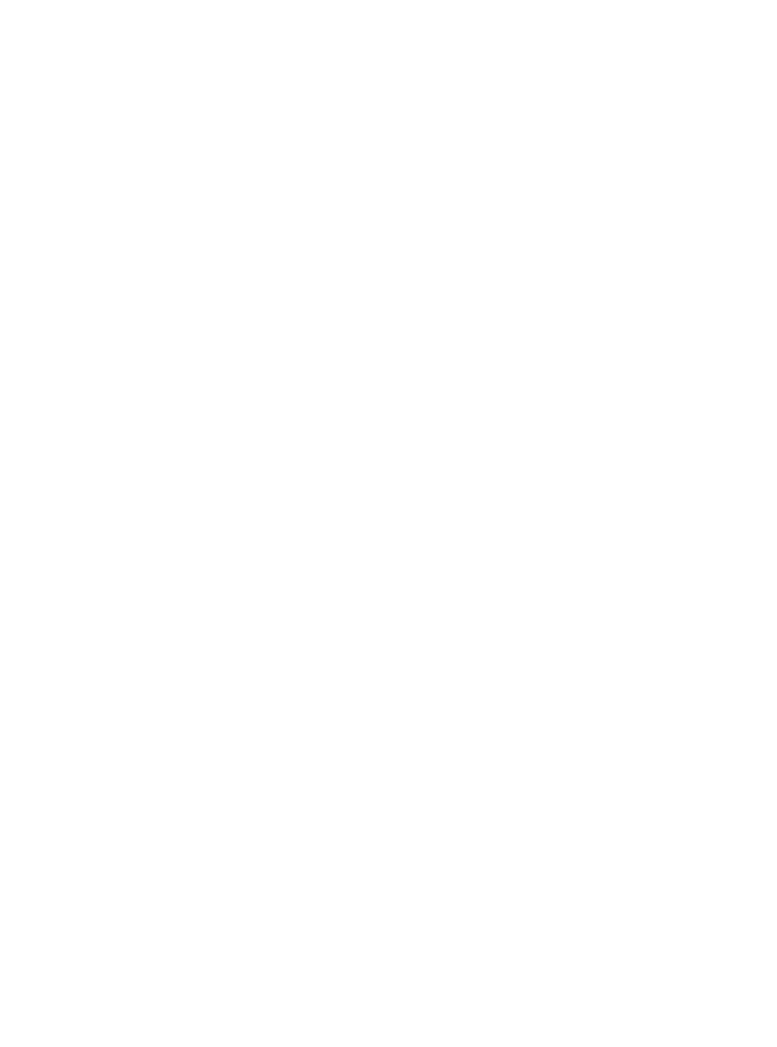
Microphone Devices and Systems 4-5
Weinberg, L.: Network Analysis and Synthesis, McGraw-Hill, New York, N.Y., 1967.
Widlar, R. J.: “A Unique Current Design for a High Performance Operational Amplifier Espe-
cially Suited to Monolithic Construction,” Proc. NEC, 1965.
Woszczyk, W. R.: “A Microphone Technique Employing the Principle of Second-Order Gradient
Unidirectionality,” presented at the 69th Convention of the Audio Engineering Society., J.
Audio Eng. Soc. (Abstracts), vol. 29, pg. 550, preprint 1800, July-August 1981.
Downloaded from Digital Engineering Library @ McGraw-Hill (www.digitalengineeringlibrary.com)
Copyright © 2004 The McGraw-Hill Companies. All rights reserved.
Any use is subject to the Terms of Use as given at the website.
Microphone Devices and Systems
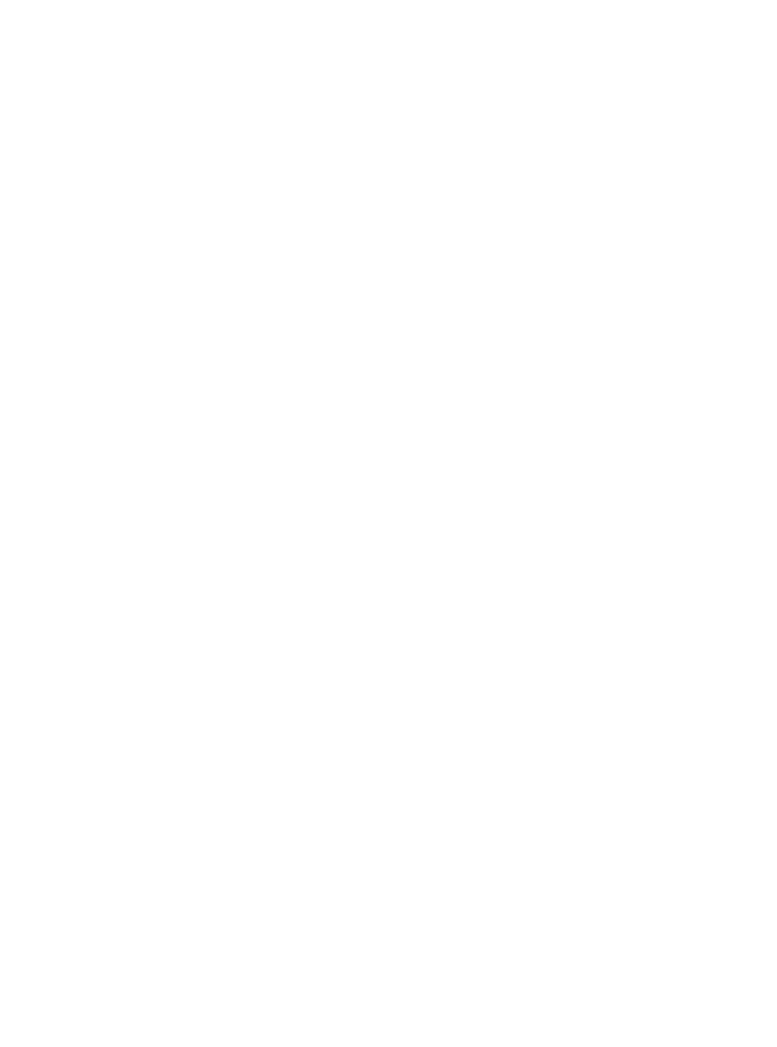
Downloaded from Digital Engineering Library @ McGraw-Hill (www.digitalengineeringlibrary.com)
Copyright © 2004 The McGraw-Hill Companies. All rights reserved.
Any use is subject to the Terms of Use as given at the website.
Microphone Devices and Systems
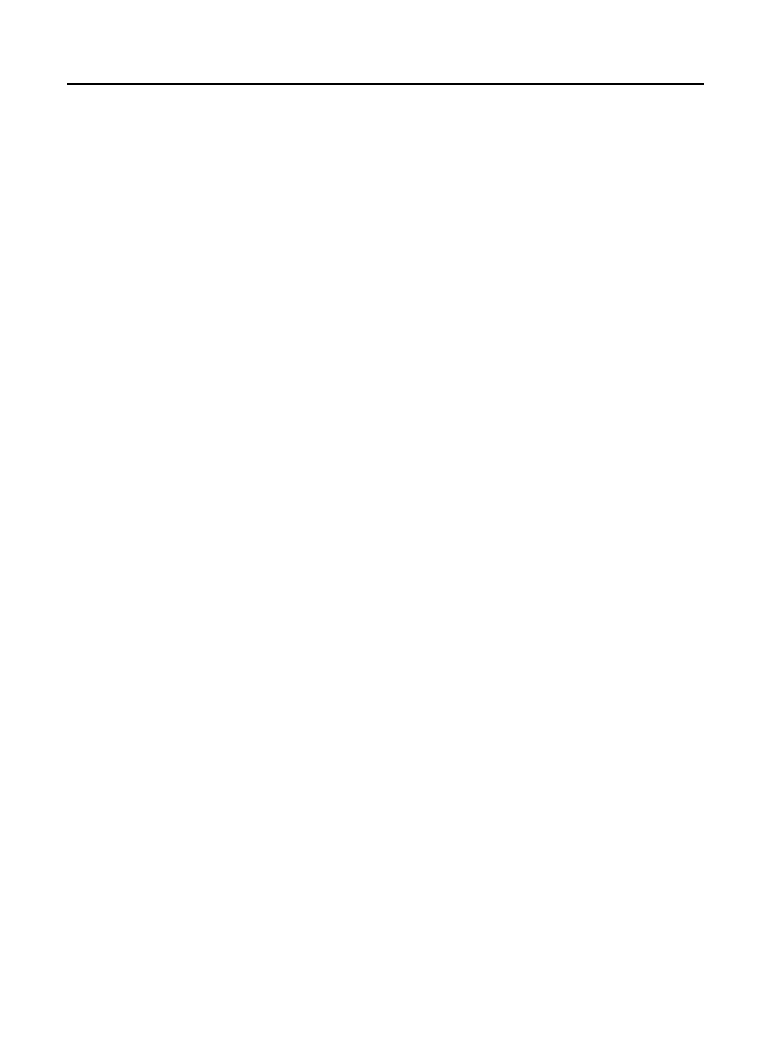
4-7
Chapter
4.1
Microphones
Jon R. Sank, Ronald D. Streicher, Wesley L. Dooley
4.1.1
Introduction
A microphone is an electroacoustic device containing a transducer which is actuated by sound
waves and delivers essentially equivalent electric waves. The classes of microphones include
pressure, pressure-gradient (velocity), combination pressure and pressure-gradient, and wave-
interference. The electrical response of a pressure microphone results from pressure variations in
the air. The directional (polar) pickup pattern is omnidirectional (nondirectional) because sound
pressure is a scalar quantity which possesses magnitude but no direction. The electrical response
of a velocity microphone results from variations in the particle velocity of the air. The polar pat-
tern is bidirectional (cosine or figure-of-eight) because particle velocity is a vector quantity
which possesses magnitude and direction. The electrical response of the combination pressure
and pressure-gradient microphone is also proportional to the particle velocity. The polar pattern
may be cardioid, hypercardioid, or of a similar cosine-function limacon shape and may be fixed
or adjustable.
A particular class of microphones may include one of the following types of transducers: car-
bon, ceramic, condenser, moving-coil, inductor, ribbon, magnetic, electronic, or semiconductor.
The functioning of various types of microphones is described in this chapter by reference to
the equivalent circuits of the acoustical and mechanical systems. The mechanical equivalent cir-
cuit is considered, for simplicity, when the discussion involves mathematical equations. In other
instances, the discussion omits mathematics, and the acoustical network affords the clearest
illustration of operating principles.
4.1.2
Pressure Microphones
A carbon microphone depends for its operation on the variation of resistance of carbon contacts.
The high sensitivity of this microphone is due to the relay action of the carbon contacts. It is
widely used in telephone communications. This is true because the high sensitivity eliminates
the need for audio amplification in a telephone set. Restricted frequency range, distortion, and
carbon noise limit the application of the carbon microphone in other than voice-communications
applications.
Downloaded from Digital Engineering Library @ McGraw-Hill (www.digitalengineeringlibrary.com)
Copyright © 2004 The McGraw-Hill Companies. All rights reserved.
Any use is subject to the Terms of Use as given at the website.
Source: Standard Handbook of Audio and Radio Engineering
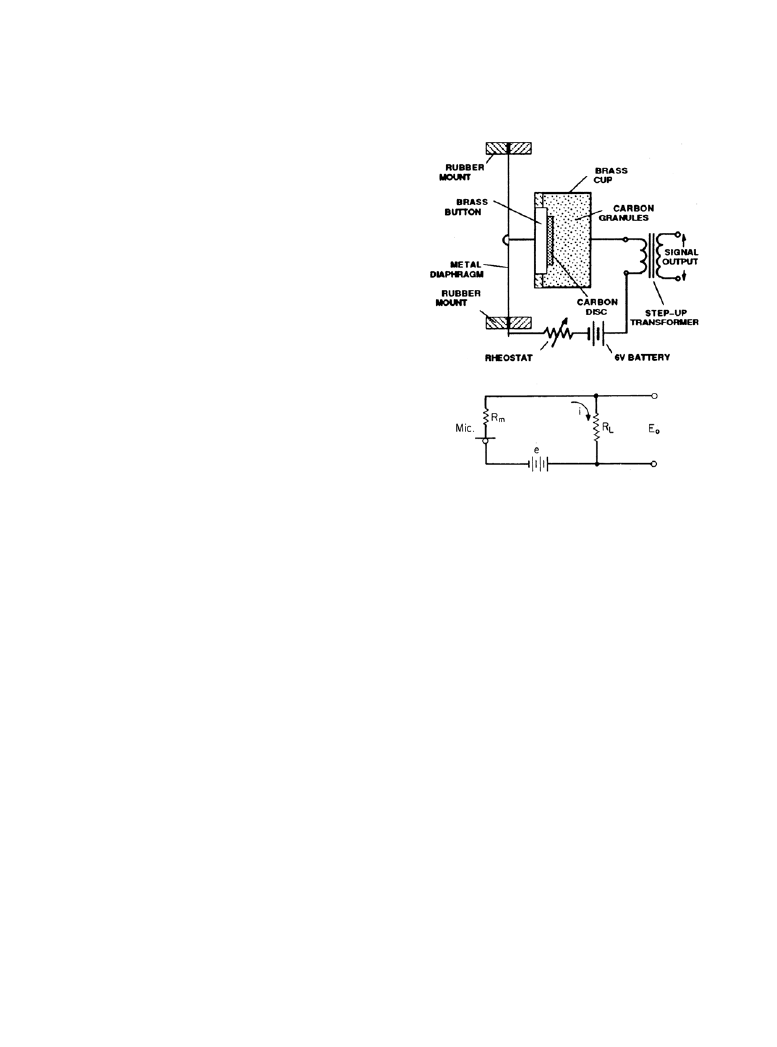
4-8 Microphone Devices and Systems
A typical single-button carbon microphone and its electric circuit are shown in Figure 4.1.1.
The carbon transducer consists of a contact cup filled with carbon granules, which are usually
made from anthracite coal [1]. The granules make contact with the electrically conductive dia-
phragm via the contact button on the diaphragm. The diaphragm is frequently made from a thin
sheet of aluminum alloy. The periodic displacement of the diaphragm causes a variation in
mechanical pressure applied to the carbon granules. This results in a periodic variation in electric
resistance from the diaphragm to the contact cup. For small displacements, the variation in resis-
tance is proportional to the displacement.
The output voltage is given by
(4.1.1)
Where:
e = dc voltage of bias source
h = constant of carbon element,
Ω/cm
x = amplitude of diaphragm, cm
ω = 2πf
f = frequency, Hz
The useful audio output is, of course, the ac component of E
0
. Equation (4.1.1) may be
expanded ([2], pg. 248) to show that the ac component consists of harmonics at f, 2f, ..., which
means that the carbon transducer has intrinsic distortion. For a limited frequency range of repro-
duction, the distortion is not objectionable.
E
0
eR
L
R
m
R
L
+
(
)
hx sin
ωt
(
)
+
----------------------------------------------------------
=
Figure 4.1.1
Carbon microphone and equiva-
lent electric circuit.
Downloaded from Digital Engineering Library @ McGraw-Hill (www.digitalengineeringlibrary.com)
Copyright © 2004 The McGraw-Hill Companies. All rights reserved.
Any use is subject to the Terms of Use as given at the website.
Microphones
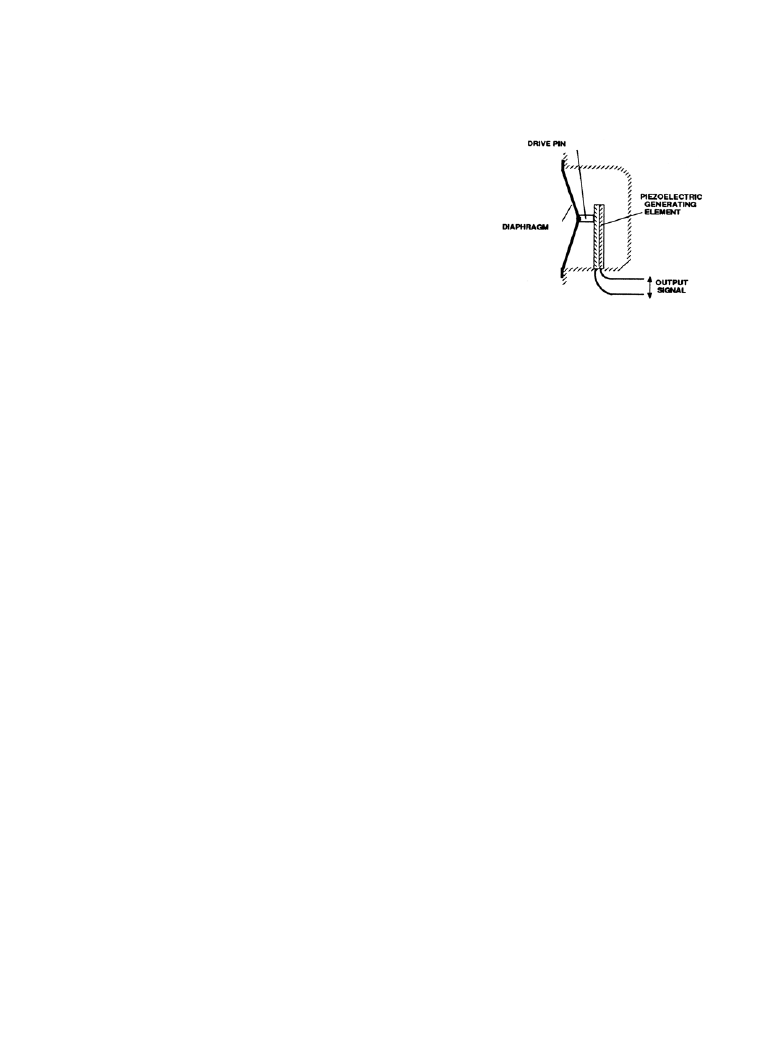
Microphones 4-9
The large second-harmonic distortion component can be eliminated through use of two car-
bon buttons in push-pull. It was used in the 1920s for broadcasting but was replaced by con-
denser, ribbon, and dynamic microphones. Although the double-button microphone has a wide-
range frequency response and low distortion, it and the single-button types suffer from carbon
compaction and carbon noise. These effects mean that the signal-to-noise ratio or dynamic range
of the microphone is variable. Repeatability of frequency response, sensitivity, and noise mea-
surements of carbon microphones are very poor.
For improved performance in telephone and speech communications, carbon microphones
have largely been replaced by dynamic, magnetic, and electret condenser microphones, which
have built-in amplifiers. These amplifiers are powered by the direct current normally provided
by the communications equipment for carbon microphones. These carbon replacements may
offer noise-canceling features as well as improved frequency response and low distortion and
noise. They are offered as replacement cartridges for telephone handsets, in replacement hand-
sets, in hand-held microphones, and in headsets.
4.1.2a
Piezoelectric Microphone
The piezoelectric microphone contains a transducer element that generates a voltage when
mechanically deformed. The voltage is proportional to the displacement in the frequency range
below the resonance of the element. Rochelle salt crystals were used prior to 1960 but were sen-
sitive to humidity and heat. Newer ceramic materials such as barium titanate and lead zirconate
titanate are more resistant to environmental extremes and have replaced the Rochelle salt crys-
tals. There are two general classifications of ceramic microphones: direct-actuated and dia-
phragm-actuated. Directly actuated transducers consist of stacked arrays of bimotph crystals or
sound cells.
Figure 4.1.2 shows the most common construction in use today for a ceramic microphone.
The element is mounted as a cantilever and actuated by the diaphragm via the drive pin. The dia-
phragm is frequently made from thin aluminum sheet, although polyester film may also be used.
The impedance of the ceramic microphone is capacitive on the order of 500 to 1000 pF. This per-
mits use of a short length of cable with only a small loss in output level. The advantage of the
ceramic microphone is that the output voltage is sufficient to drive a high-impedance input of an
amplifier directly. The frequency response (with a very high input resistance) is uniform from a
very low frequency up to the transducer resonance, which may be situated at 10,000 Hz or
higher. The sensitivity and the frequency response are stable with time and over a wide range of
Figure 4.1.2
Typical construction of a ceramic microphone.
Downloaded from Digital Engineering Library @ McGraw-Hill (www.digitalengineeringlibrary.com)
Copyright © 2004 The McGraw-Hill Companies. All rights reserved.
Any use is subject to the Terms of Use as given at the website.
Microphones