ВУЗ: Не указан
Категория: Не указан
Дисциплина: Не указана
Добавлен: 02.10.2020
Просмотров: 1297
Скачиваний: 6
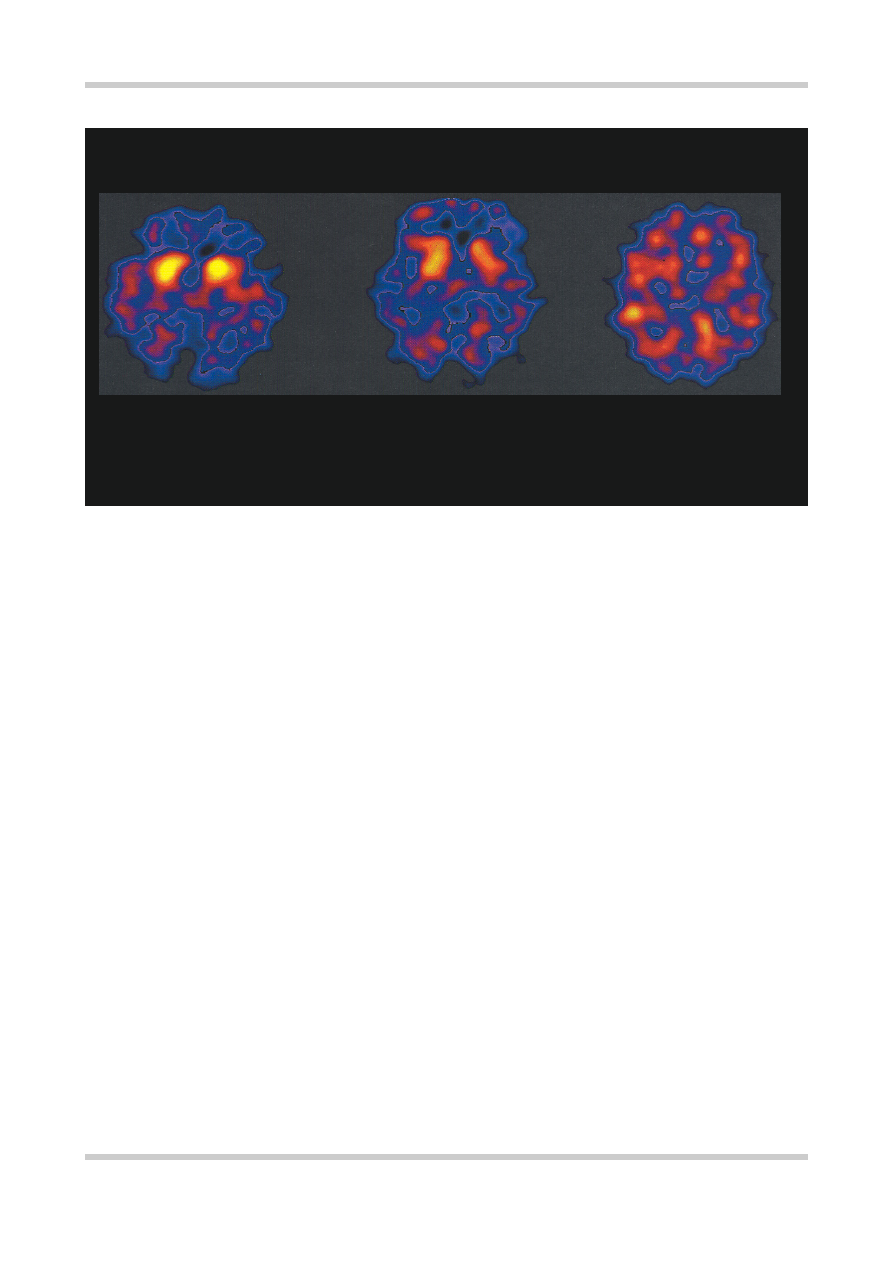
©2002 CRC Press LLC
another showing no change
5
. Although the
majority of subsequent studies with PET or SPET
have found no significant difference in striatal D
2
receptor density between controls and patients
with schizophrenia, in a recent meta-analysis of
studies carried out between 1986 and 1997, a
small but significant elevation of D
2
receptors was
noted in patients with schizophrenia
5
. However,
the variability of D
2
receptor density is high both
in controls and patients with schizophrenia, and it
has been argued that the small magnitude of the
effect (approximately a 12% increase), and the
possibility that any change reflects alteration in
the baseline synaptic dopamine, means that there
are no real functional differences in the density of
striatal D
2
receptors in schizophrenia (reviewed in
reference 5).
Despite these equivocal findings in drug-naive
patients, emission tomography has reinforced the
accepted theory that antipsychotic efficacy is
related to D
2
, with 65% blockade a putative
threshold for antipsychotic response
6
. Nonethe-
less, the situation appears very complex in that
non-responders to antipsychotics still show high
levels of striatal D
2
blockade
7,8
. Furthermore, the
highly effective atypical antipsychotic clozapine
produces far less striatal D
2
blockade than typical
antipsychotics
7–9
, often below the putative 65%
threshold (
Figure 4.2
). These findings have yet to
be fully explained.
Extrapyramidal side-effects (EPS) have also
been studied and a threshold of 80% D
2
occup-
ancy has been repeatedly shown to be necessary to
produce EPS
6
(
Figure 4.3
). It may be that high
levels of 5-HT
2A
receptor blockade, as seen with
Healthy volunteer
High D
2
receptor availability =
no occupancy
Clozapine treated
patient with schizophrenia
Medium D
2
receptor availability =
medium (~60%) occupancy
Typical antipsychotic-treated
patient with schizophrenia
Low D
2
receptor availability =
high (80%+) occupancy
[123I]
IBZM SPET SCANS OF STRIATAL D
2
RECEPTOR OCCUPANCY
Figure 4.2
Three single photon emission tomography (SPET), scans of striatal D
2
and D
2
-like receptor availability at the level
of the basal ganglia. In the scan on the left from a healthy volunteer there is no receptor occupancy and therefore 100%
receptor availability for the binding of the D
2
receptor tracer
[123I]
IBZM. The scan on the right is from a patient with
schizophrenia receiving a typical antipsychotic. The bright areas from the left hand scan indicating high receptor density are
not evident on the right-hand scan as the antipsychotic is occupying the majority of the receptors and preventing the tracer
from binding. Unfortunately despite this high level of occupancy this patient has failed to respond to treatment. The central
scan is from a patient receiving clozapine, although the striatum are not as ‘bright’ as in the healthy volunteer they are visible.
This scan indicates intermediate occupancy of the receptors by clozapine. Importantly the patient with the intermediate
occupancy has responded to treatment. Studies such as this one, when performed in larger groups, indicate that the simple
dopamine hypothesis suggested by the data in
Figure 4.1
needed refining. Figure reproduced with permission from Pilowsky
LS, Costa DC, Ell PJ,
et al.
Clozapine, single photon emission tomography, and the D
2
dopamine receptor blockade
hypothesis of schizophrenia.
Lancet
1992;340:199–202
©2002 CRC Press LLC
RELATIONSHIP BETWEEN D2 RECEPTOR
OCCUPANCY EPS AND RESPONSE
100%
50%
0
% Striatal D
2
Receptor Occupancy
Dose of antipsychotic
No EPS
ineffective
potentially
effective &
No EPS
potentially
effective but
high EPS risk
Clinical effect
Figure 4.3
Illustration of the relationship between clinical effectiveness, extrapyramidal side-
effects (EPS) and D
2
receptor occupancy. With the exception of clozapine-induced D
2
occupancy,
below 60% striatal D
2
occupancy produced by an antipsychotic medication is likely to be
associated with treatment non-response, whilst occupancies above 80% are likely to be associated
with a high risk of EPS. Once a patient is receiving a dose of an antipsychotic likely to be
producing an occupancy between 60% and 80% there is little to be gained, in terms of treatment
response, by increasing the dose of the antipsychotic further as the patient will almost certainly
develop EPS. Figure after references 6, 7 and 51
Figure 4.4
The effect of amphetamine (0.3 mg/kg) on
[123I]
IBZM binding in healthy control subjects and
untreated patients with schizophrenia.
[123I]
IBZM is a
tracer for D
2
receptors which allows
in vivo
measurement of D
2
receptor availability (or binding
potential) in humans using single photon emission
tomography (SPET). The y-axis shows the percentage
decrease in
[123I]
IBZM binding potential induced by
amphetamine, which is a measure of the increased
occupancy of D
2
receptors by dopamine following the
challenge. Thus, these results indicate that, when
challenged with amphetamine, patients with
schizophrenia release more dopamine than do healthy
controls. Figure reproduced with permission from
Laruelle M, Abi-Dargham A, Gil R,
et al.
Increased
dopamine transmission in schizophrenia: relationship
to illness phases [Review].
Biol Psychiatry
1999;
46:56–72
50
0
10
20
30
40
[123
1]IBZM displacement by
amphetamine (% baseline)
p < 0.001
Controls Schizophrenics
DOPAMINE RELEASE BY AMPHETAMINE
most of the newer antipsychotics, may be
protective against EPS by altering this threshold
(reviewed in reference 10).
Recent neurochemical imaging studies have
indicated that people with schizophrenia have an
increased sensitivity of their dopaminergic
neurones to amphetamine challenge
11
(
Figure
4.4
). Thus, it may be that in response to ‘stress’
such people will over-release dopamine and this
may drive psychosis.
There are currently five types of dopamine
receptors identified in the human nervous system:
D
1
to D
5
. D
1
and D
5
receptors are similar in that
they both stimulate the formation of cAMP by
activation of a stimulatory G-coupled protein. D
2
to D
4
receptors act by activating an inhibitory G-
protein, thereby inhibiting the formation of
cAMP. D
2
receptors are more ubiquitous than D
3
or D
4
receptors. D
3
receptors are differentially
situated in the nucleus accumbens (one of the
septal nuclei in the limbic system) and D
4
receptors are especially concentrated in the frontal
cortex (
Figure 4.5
). There are a number of
different dopaminergic pathways or tracts (
Figure
4.6
). The nigrostriatal tract projects from the
substantia nigra in the midbrain to the corpus
striatum. This tract primarily has a role in motor
control, although the ventral striatum has a role in
reward- and goal-directed behaviors. Degeneration
of the cells in the substantia nigra leads to
idiopathic Parkinson’s disease, and it is by blocking
the dopamine receptor at the termination of this
pathway that the parkinsonian side-effects of
classical antipsychotics arise. The mesolimbic/
mesocortical tract has its cell bodies in the ventral
tegmental area adjacent to the substantia nigra.
This tract projects to the limbic system and
neocortex in addition to the striatum. This
Figure 4.5
There are currently five types of dopamine receptor identified in the human nervous system, D
1
to D
5
.
D
1
and D
5
receptors are similar in that they both stimulate the formation of cAMP by activation of a stimulatory
G-coupled protein. D
1
and D
5
are therefore known as D
1
-like receptors. D
2
to D
4
receptors activate an inhibitory
G-protein, thereby inhibiting the formation of cAMP. They are collectively known as D
2
-like receptors. D
2
receptors are more ubiquitous than D
3
or D
4
receptors. D
3
receptors are differentially situated in the nucleus
accumbens (one of the septal nuclei in the limbic system) and D
4
receptors are especially concentrated in the
frontal cortex
CLASSIFICATION OF DOPAMINE RECEPTORS
Caudate-
putamen
N. accumbens
Olfactory
tubercule
Hippocampus
Hypothalamus
Olfactory
tubercule
Hypothalamus
N. accumbens
Cerebellum
Frontal
cortex
Medulla
Midbrain
Caudate-
putamen
N. accumbens
Olfactory
tubercule
Dopamine
D
1
receptor
family
D
1
D2 D3 D4
D
5
D
2
receptor
family
©2002 CRC Press LLC
dopaminergic innervation supplies fibers to the
medial surface of the frontal lobes and to the
parahippocampus and cingulate cortex, the latter
two being part of the limbic system. Because of
this anatomic representation it is thought that this
tract is where antipsychotic medication exerts its
beneficial effect. The third major pathway is
termed the tuberoinfundibular tract. The cell
62
Figure 4.6
Representation of the primary dopamine-containing tracts in the human brain. The nigrostriatal tract is
primarily involved in motor control, but also in reward- and goal-directed behavior. Blockade of D
2
receptors here
produces some of the antipsychotic effects of antipsychotics, but high levels of blockade (> 80%) produce
parkinsonian side-effects. Blockade of D
2
receptors in the tuberoinfundibular pathway increases plasma prolactin.
It is thought that it is the blockade of D
2
and D
2
-like receptors in the mesolimbic and mesocortical tracts that
underlies the primary antipsychotic effects of all currently available antipsychotics
THE DOPAMINERGIC PATHWAYS
A Substantia nigra
B Ventral tegmental area
C To amygdala
D Tuberoinfundibular DA system
E Nucleus accumbens
(ventral striatum)
F To the striatum (caudate nucleus,
putamen and globus pallidus)
G Frontal cortex
E
F
D
A
G
B
C
bodies for this tract reside in the arcuate nucleus
and periventricular area of the hypothalamus.
They project to the infundibulum and the anterior
pituitary. Dopamine acts within this tract to
inhibit the release of prolactin. The blockade of
these receptors by antipsychotics removes the
inhibitory drive from prolactin release and leads
to prolactinemia.
Figure 4.7
Dopamine is synthesized in
a common pathway with
norepinephrine. In the pathway shown,
tyrosine hydroxylase is the rate-limiting
enzyme. Dopamine
β
-hydroxylase is
only found in noradrenaline neurons in
the CNS
Phenylalanine
Tyrosine
tyrosine
hydroxylase
(pterin cofactor)
Dopa
decarboxylase
(pyridoxal cofactor)
Dopamine
ȋ
-hydroxylase
(copper containing enzyme)
Ascorbate and O
2
required
Dopa
Dopamine
Norepinephrine
Rate
limiting
step
H
H
C CH
COOH
NH
2
H
H
C CH
COOH
NH
2
H
H
C CH
HO
HO
HO COOH
NH
2
H
H
C CH
2
HO
HO
NH
2
OH
H
C CH
2
HO
HO
NH
2
DOPAMINE SYNTHESIS
©2002 CRC Press LLC
Figure 4.8
Dopamine is
metabolized by two enzymes.
One, monoamine oxidase type B
(MAO-B), is intraneuronal, and the
other, catechol-
O
-methyl
transferase (COMT), is extraneur-
onal. The primary metabolite of
dopamine is homovanillic acid
Dopamine
Catechol-O-methyl
transferase (COMT)
Monoamine oxidase
type B (MAO-B)
Intraneural
Homovanillic acid
(HVA)
DOPAMINE METABOLISM
Extraneural
Dopamine is synthesized as part of the com-
mon pathway for catecholamines (
Figure 4.7
).
Dopamine is metabolized by two enzymes; one,
monoamine oxidase type B (MAO-B), is
intraneuronal, and the other, catechol-O-methyl
transferase (COMT), is extraneuronal. The prim-
ary metabolite of dopamine is homovanillic acid
(HVA;
Figure 4.8
).
Serotonin
The serotonergic hypothesis of schizophrenia
predates the dopaminergic hypothesis and stems
from the finding by Woolley and Shaw in 1954
12
that the hallucinogen LSD acted via serotonin.