ВУЗ: Казахская Национальная Академия Искусств им. Т. Жургенова
Категория: Книга
Дисциплина: Не указана
Добавлен: 03.02.2019
Просмотров: 21711
Скачиваний: 19
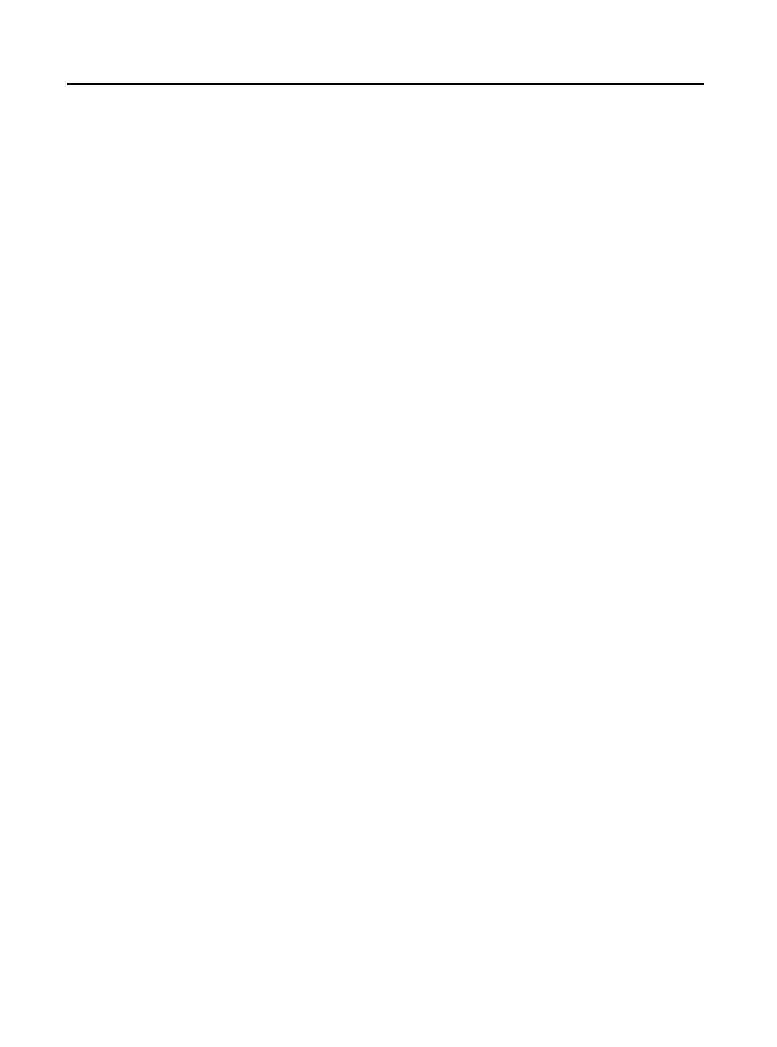
9-47
Chapter
9.4
Magnetic Tape
Robert H. Perry
9.4.1
Introduction
Magnetic tape includes a multiplicity of products used for magnetic recording, all consisting of a
magnetizable medium on a flexible substrate. Because of the great variety of machine types and
recording formats in use and being developed, magnetic tape is designed and produced with
widely different magnetic media, widths, thicknesses, lengths, and other properties optimized for
each application. Media are used in either strip form in reels, cartridges, cassettes, and cards or in
discs of different diameters. Similar technologies are used to produce all these products.
9.4.2
Basic Construction
Magnetic tape consists of a magnetic film or coating supported by a flexible substrate, or base
film, which in many applications is coated on the back with a nonmagnetic coating (Figure
9.4.1).
Backcoatings are used primarily in the most demanding tapes, such as professional and some
consumer video, professional audio, instrumentation, and data products, where special winding
and handling characteristics are required. This coating contains a conductive pigment, usually
carbon black, which reduces buildup of static charge and therefore minimizes the accumulation
of dirt and debris on the tape, factors which can cause drop-outs, or loss of signal with attendant
loss of stored information. The backcoating also provides better frictional characteristics than
raw base film does, and air is more easily eliminated from adjacent layers during winding. This
reduces the tendency of the tape to cinch or form pop strands, and there is less likelihood of
uneven stacking, edge damage, and creasing of the tape.
The base film is an integral and significant part of the whole tape system and is largely
responsible for its mechanical strength and stability. Other factors such as stiffness and surface
smoothness have a profound influence on tape performance in many applications, and base films
having the proper characteristics for a given application must be carefully selected.
The principal substance used in the great preponderance of magnetic tapes today is poly(eth-
yleneterephthalate), or simply polyester, abbreviated PET. PET has an excellent combination of
properties including chemical stability and mechanical properties, such as tensile strength, elon-
Downloaded from Digital Engineering Library @ McGraw-Hill (www.digitalengineeringlibrary.com)
Copyright © 2004 The McGraw-Hill Companies. All rights reserved.
Any use is subject to the Terms of Use as given at the website.
Source: Standard Handbook of Audio and Radio Engineering
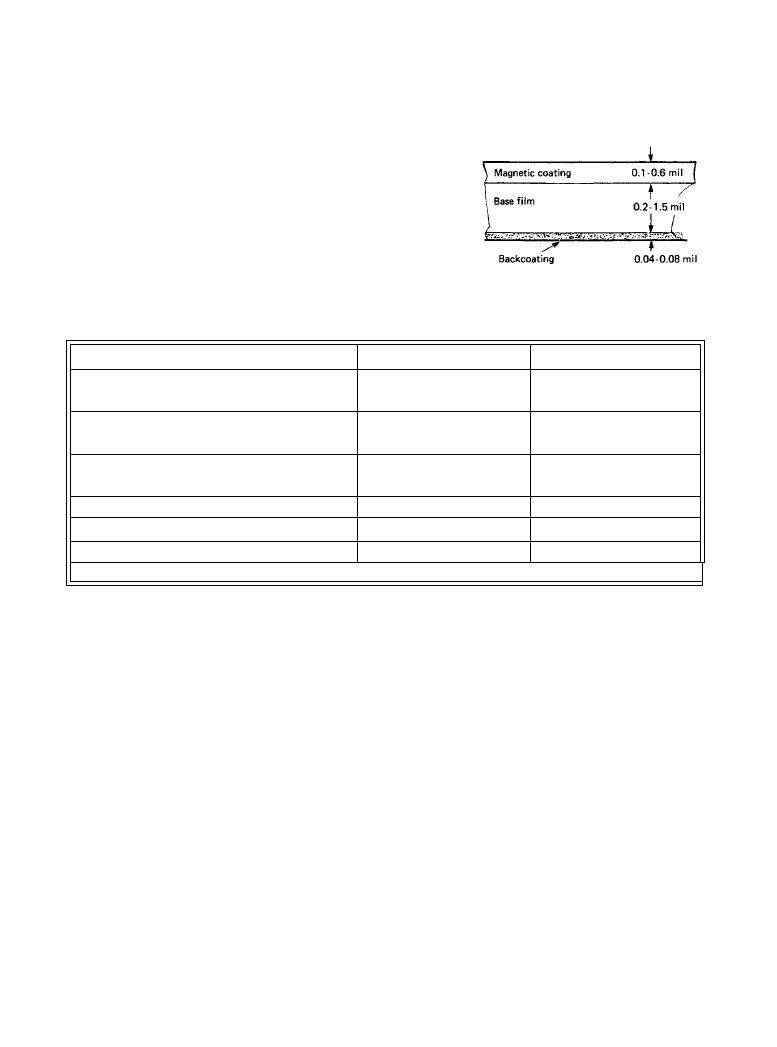
9-48 Audio Recording Systems
gation and modulus, tear resistance, availability, and cost. Some typical properties are shown in
Table 9.4.1.
Many different types and grades of PET are on the market for both magnetic tape-related and
unrelated applications. In all cases, mechanical strength in the plastic film is achieved during its
manufacture by a process of biaxial, and sometimes uniaxial, orientation of polymer chains in
the hot film after extrusion of the melt. Biaxial orientation is achieved by stretching in both the
machine and the transverse directions, and the resulting film has a balance of properties in the
two directions. Balanced film is adequate for many magnetic tape applications, especially those
employing gauge thicknesses greater than 0.5 mil (0.0127 mm). In thinner gauges greater resis-
tance to stretching is needed, and PET is used that is tensilized, i.e., oriented by drawing addition-
ally in the machine direction.
Base films for magnetic tape range in thickness from about 0.2 to 1.5 mils (3 mils for flexible
disks). They are employed by tape manufacturers in widths ranging from 12 to 60 in (0.3 to 1.5
m) and in lengths up to 15,000 ft (4572 m). The base-film manufacturer must ensure that the
base film has the right balance of surface smoothness for recording performance and roughness
for runability in the coating and processing steps. Small-particle-size, inorganic additives are
incorporated in the PET to provide slip properties in film that would be otherwise unmanage-
able. These surface asperities must be critically controlled, especially for short-wavelength
Figure 9.4.1
Magnetic-tape base-film and coating
thickness.
Table 9.4.1 Physical Properties of Poly(ethyleneterephthalate) Base
Property
Balanced
Tensilized
Tensile strength, lb/in
2
N/m
2
25,000
172.38
×
10
6
40,000
275.8
×
10
6
Force to elongate 5%, lb/in
2
N/m
2
14,000
96.53
×
10
6
22,000
151.69
×
10
6
Elastic modulus, lb/in
2
N/m
2
550,000
3.79
×
10
9
1,100,000
7.58
×
10
9
Elongation,%
130
40
Thermal coefficient of linear expansion, per deg C
1.7
×
10
–5
1.7
×
10
–5
Shrinkage at 100°C, % (per 30 min interval)
0.4
2.5
Note: Measurements in machine direction
Downloaded from Digital Engineering Library @ McGraw-Hill (www.digitalengineeringlibrary.com)
Copyright © 2004 The McGraw-Hill Companies. All rights reserved.
Any use is subject to the Terms of Use as given at the website.
Magnetic Tape
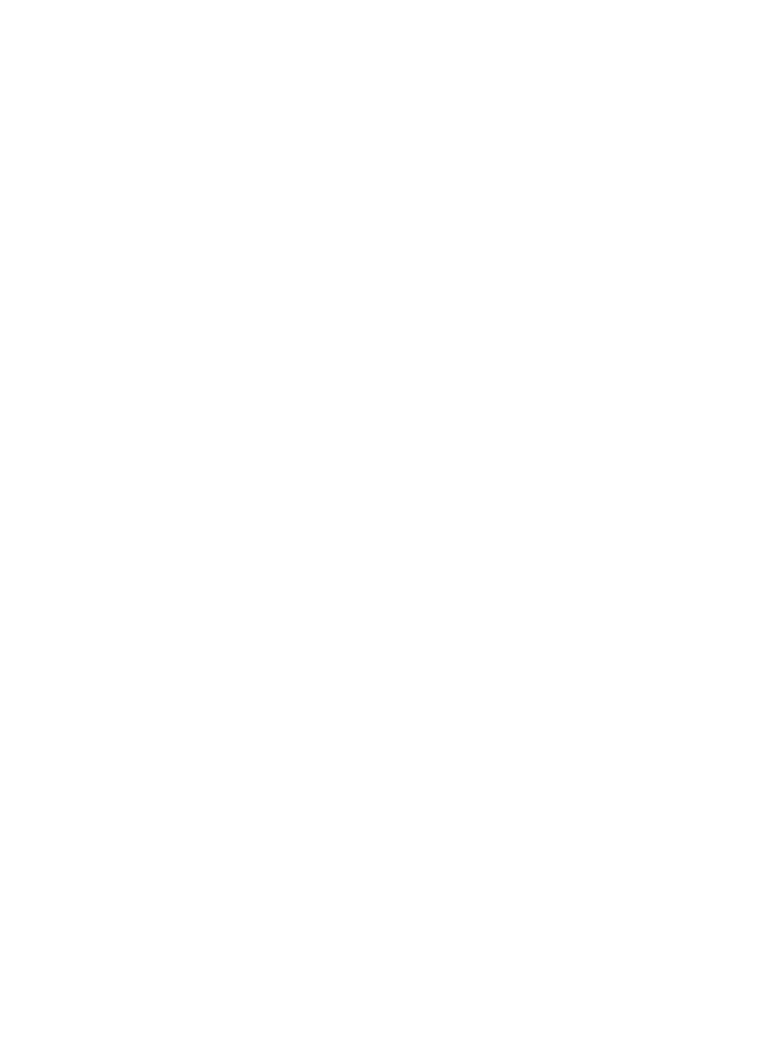
Magnetic Tape 9-49
recording applications, since the base-film surface-roughness profile can be carried—to a
degree—through the magnetic coating and reflected in the tape-surface roughness. An asperity
of 10
µin, for example, in a typical 100-µin-wavelength video recording can result in a loss of
signal of 5.5 dB due to head-to-tape separation, as seen from the Wallace formula:
Spacing loss (dB) = 54.6 d/
λ
(9.4.1)
where d = the head-tape spacing and
λ = the wavelength.
9.4.2a
Magnetic Coating
There are two types of magnetic coatings used in magnetic tape. Most of them use magnetic par-
ticles bound in a matrix of organic, polymeric binder that is applied to the substrate from a dis-
persion in solvents. Other types are made by vapor deposition of thin films of metal alloys.
Most magnetic coatings contain a single layer, although some tapes are made with dual-layer
magnetic coatings having different coercivities and are designed to have flat response over a
range of frequencies. Magnetic-tape performance is a function of both the formulation of ingre-
dients in the coating and the process by which the coating is applied and processed. The most
important component in the formulation is the magnetic material itself.
Magnetic Materials
A wide variety of single-domain magnetic particles is used having different properties, depend-
ing on the electrical requirements of each tape application. Retentivities range from about 1000
to 3000 G, and coercivities range from about 300 to 1500 Oe. Size and shape are important
because they relate to how well the particles pack in the coating; the signal-to-noise ratio achiev-
able is proportional to the number of particles per unit volume in the coating. The length of the
particles is about 8 to 40
µin (0.2 to 1.0 µm), and they are acicular with aspect ratios of 5/1 to 10/
1. Acicularity makes the particles magnetically anisotropic, and thus it governs magnetic proper-
ties not inherent in the material. In general, magnetic pigments are loaded to as high a level as
possible commensurate with retention of desirable physical properties and avoidance of shed-
ding. The limiting factor is the amount of pigment the binder can retain without loss of cohesion
and, hence, durability.
There are four basic types of magnetic particles used in tape:
•
γ -ferric oxide
•
Doped iron oxides
•
Chromium dioxide
•
Metallic particles, which usually consist of elemental iron, cobalt, and/or nickel
γ -ferric oxide has been by far the most widely used material (Hc 300 to 360 Oe) and is useful
for many of the lower-energy applications in which the ultimate in recording density or short-
wavelength recording capability is not required. The sequence of steps used in the commercial
production of
γ -ferric oxide is as follows:
Downloaded from Digital Engineering Library @ McGraw-Hill (www.digitalengineeringlibrary.com)
Copyright © 2004 The McGraw-Hill Companies. All rights reserved.
Any use is subject to the Terms of Use as given at the website.
Magnetic Tape
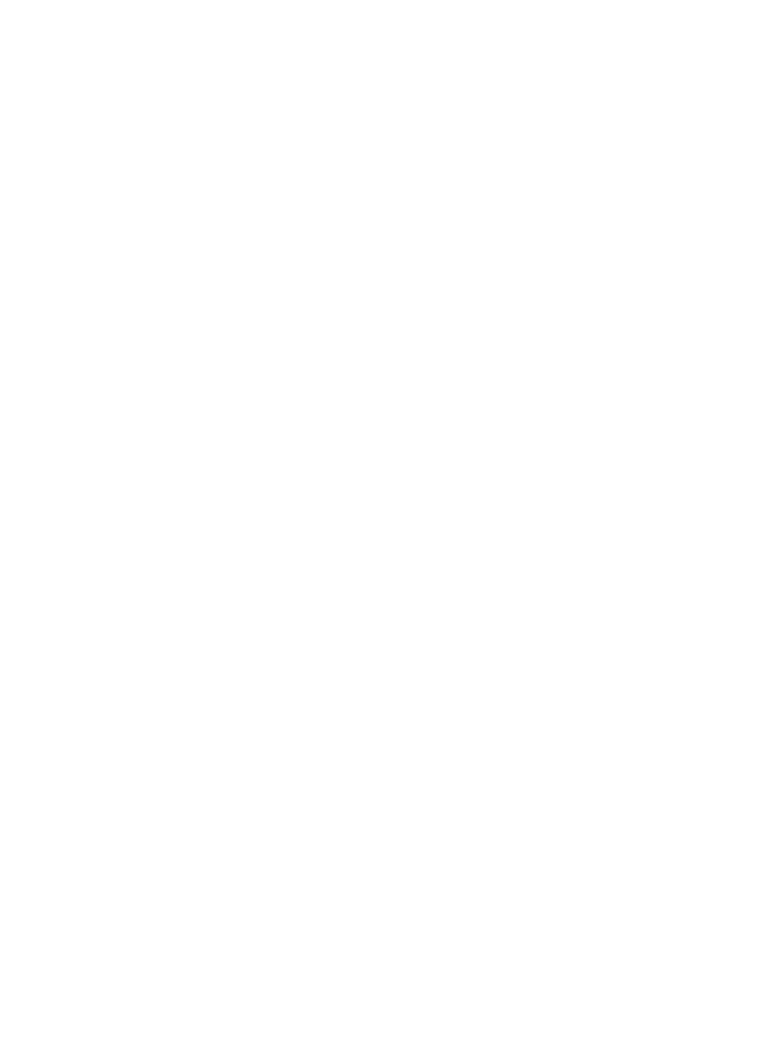
9-50 Audio Recording Systems
•
Precipitation of seeds of
α-FeOOH (goethite) from solutions of scrap iron dissolved in sulfu-
ric acid, or from copperas (ferrous sulfate obtained as a by-product from titanium dioxide
manufacture)
•
Growth of more goethite on the seeds
•
Dehydration to
α-Fe
2
0
3
(hematite)
•
Reduction to Fe
3
0
4
(magnetite)
•
Oxidation to
γ -ferric oxide (maghemite)
An improved
γ -ferric oxide is produced starting with ferrous chloride rather than ferrous sul-
fate and precipitating
γ -FeOOH (lepidocrocite) rather than α-FeOOH in the initial step.
Cobalt doping of iron oxide affords particles with higher coercivities (500 to 1200 Oe). The
older process involves precipitation of cobaltous salts with alkali in the presence of yellow iron
oxide (
α-FeOOH), dehydration, reduction to cobalt-doped magnetite, and oxidation to cobalt-
doped magnetite containing varying amounts of FeO. The resulting particles have cobalt ions
within the lattice of the oxide, and they exhibit a marked magnetocrystalline anisotropy. This
gives rise both to a strong temperature dependence of the coercivity and to magnetostrictive
effects, which can cause problems of greatly increased print-through, increased noise, and loss of
output resulting from stress on the tape through head contact. Improved stability can be achieved
by using other additives, such as zinc, manganese, or nickel, with cobalt.
Epitaxial cobalt-doped particles can be used that largely overcome these problems because
cobalt ion adsorption is limited to the surface of the oxide. Epitaxial particles have superseded
lattice-doped particles in most applications.
Chromium dioxide provides a range of coercivities similar to that of cobalt-doped iron oxide
(450 to 650 Oe) and possesses a slightly higher saturation magnetization, that is, 80 to 85 emu/g
compared with 70 to 75 emu/g for
γ -ferric oxide. It has uniformly good shape and high acicular-
ity and lacks voids and dendrites, factors that account for the excellent rheological properties of
coating mixes made with it. Its low Curie temperature (128°C) has been exploited in thermal
contact duplication, a process which was largely developed in the late 1960s but because of prob-
lems in obtaining high-quality duplicates was not commercialized.
A disadvantage of chromium dioxide is its abrasiveness, which can cause excessive head
wear. Also, it is chemically less stable than iron oxide, and under conditions of high temperature
and humidity it can degrade to nonmagnetic chromium compounds, resulting in loss of output of
the tape. Chromium dioxide and cobalt-doped iron oxide yield tapes having 5 to 7 dB higher S/N
ratio than those made from
γ -ferric oxide.
The presence of metallic particles results in tapes that have a 10 to 12-dB higher S/N ratio
than those made from
γ -ferric oxides because of their much higher saturation magnetization
(150 to 200 emu/g), retentivity (2000 to 3000 G), coercivity (1000 to 1500 Oe), and smaller par-
ticle size, These factors, together with a square shape of the hysteresis loop, permit recording at
shorter wavelengths with less self-erasure. Thus, recordings can be made at slower speeds with-
out sacrifice in dynamic range, and higher bit-packing densities can be achieved.
Metallic particles are made by several different kinds of processes, the more important com-
mercial ones being reduction of iron oxide with hydrogen, and chemical reduction of aqueous
ferrous salt solutions with borohydrides. Metallic particles are more difficult to disperse than
iron oxides because of their smaller size and higher remanence, and they are highly reactive. Pro-
cesses such as partial oxidation of the surface or treatment with chromium compounds are used
Downloaded from Digital Engineering Library @ McGraw-Hill (www.digitalengineeringlibrary.com)
Copyright © 2004 The McGraw-Hill Companies. All rights reserved.
Any use is subject to the Terms of Use as given at the website.
Magnetic Tape
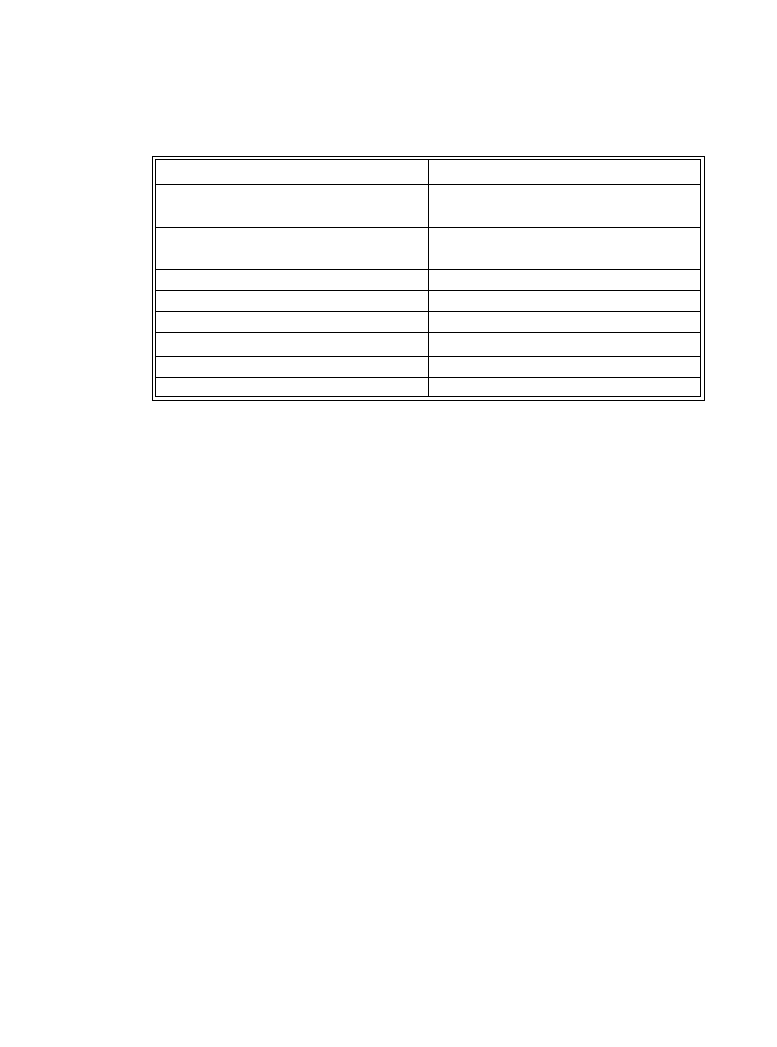
Magnetic Tape 9-51
in their preparation to stabilize them for handling during tape manufacture. The corresponding
tapes are more stable, but their susceptibility to corrosion at an elevated temperature and humid-
ity is a disadvantage.
Magnetic-tape manufacturers have also developed products consisting of thin films (100 to
150 nm) of metal alloys deposited on the substrate under vacuum or by sputtering. The retentiv-
ity of these tapes (1.2
× 10
4
G) is almost an order of magnitude higher than that of
γ -ferric
oxides, with a corresponding increase in recording density.
In other areas, research is being devoted to very small, isotropic particles, which have aspect
ratios of 1/1 to 2/1, because of advantages that can be taken of magnetization vectors in more
than one direction, i.e., vertical as well as longitudinal recording, and because of the increased
number of particles that can be packed in a coating per unit volume. Particles having the shape of
rice grains have been used with success.
9.4.2b
Binders
Binders must be capable of holding the magnetic pigment together in a flexible film that adheres
to the base film with a high degree of toughness and chemical stability, and with thermoplastic
properties enabling the pigmented film to be compacted to give smooth surfaces. It should also
be soluble in suitable solvents. These requirements are not met by many substances available
today for producing magnetic tapes.
Polyurethanes, either used as such or prepared in situ, represent the most important class of
polymers for this purpose because of their affinity for pigments, their toughness and abrasion
resistance, and their availability in soluble forms. Of the two types in use, poly(esterurethanes)
are preferred over poly(etherurethanes) because of their superior mechanical properties in tape.
Some physical properties of a typical poly(esterurethane) are shown in Table 9.4.2.
Other polymers can be used alone or in combination with one or two other polymers to obtain
the desired properties. Although a great many types are claimed in the patent literature, the other
Table 9.4.2 Physical Properties of a Poly(esterurethane)
Parameter
Value
Tensile strength, lb/in
2
N/m
2
8000
55.16
×
10
6
Stress at 100% elongation, lb/in
2
N/m
2
300
2.07
×
10
6
Ultimate elongation,%
450
Glass transition temperature, deg C
12
Hardness, shore A
76
Density, g/cm
3
1.17
Viscosity at 15% solids/tetrahydrofuron, cP
800
Pa
⋅
s
0.8
Downloaded from Digital Engineering Library @ McGraw-Hill (www.digitalengineeringlibrary.com)
Copyright © 2004 The McGraw-Hill Companies. All rights reserved.
Any use is subject to the Terms of Use as given at the website.
Magnetic Tape