ВУЗ: Казахская Национальная Академия Искусств им. Т. Жургенова
Категория: Книга
Дисциплина: Не указана
Добавлен: 03.02.2019
Просмотров: 21621
Скачиваний: 19
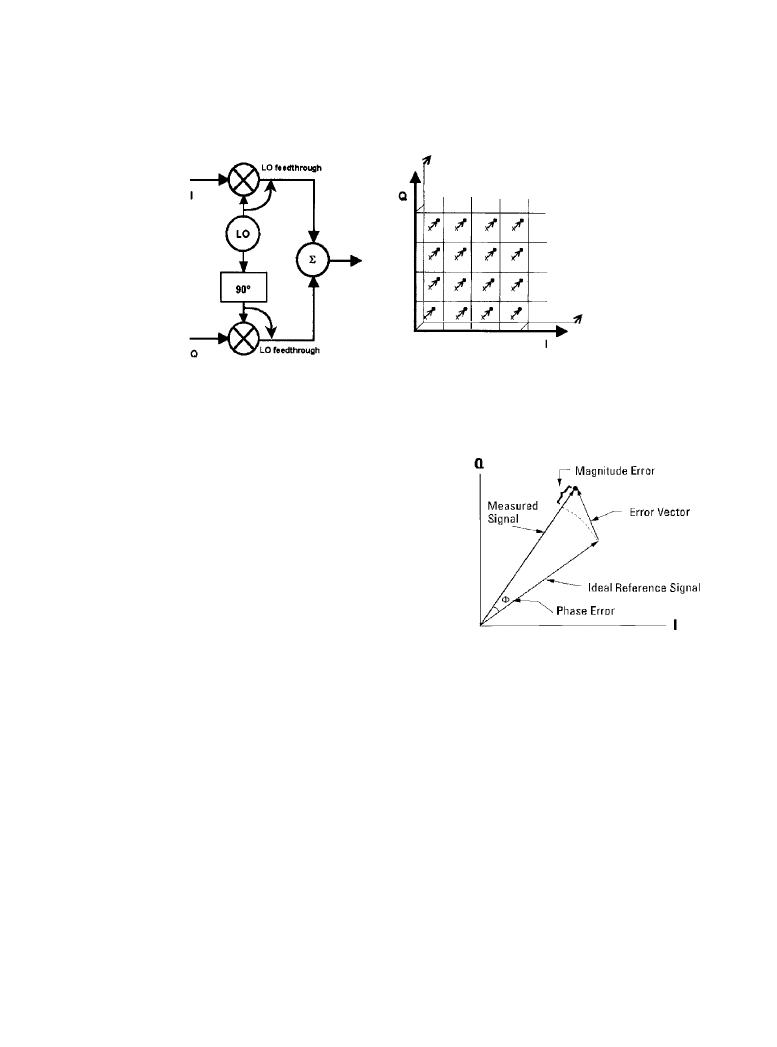
Receiver Characteristics 12-23
cuit and a low-pass negative-feedback voltage is derived to maintain a relatively constant signal
level.
A block diagram of a dual AGC loop system is illustrated in Figure 12.1.11. One loop is
driven by first IF energy that is band-limited, and the other loop is driven by second IF energy
that is band-limited by optional second IF filters. The first loop controls a PIN diode pi attenua-
tor ahead of the first mixer. The second loop controls the second IF amplifier stages. In this
design, a microprocessor adjusts the time constants of both loops so that time delays introduced
by the filters do not cause AGC oscillation.
A number of tests of gain control characteristics are customarily required. MGC may be
designed to control gain continuously or in steps. It is important that the steps be small enough
that operators do not detect large jumps as they adjust the gain. Because the gain must be con-
trolled over a very wide range, the MGC is easiest to use if it tends to cause a logarithmic varia-
tion. Usually, the testing of the MGC is confined to establishing that a specified range of gain
control exists and measuring the degree of decibel linearity versus control actuation.
Figure 12.1.9
Effect of LO-feedthrough-based
IQ offset on data signal phase constellation. (Cour-
tesy of Rohde & Schwarz.)
Figure 12.1.10
The concept of error vector magni-
tude.(
Courtesy of Hewlett-Packard.))
Downloaded from Digital Engineering Library @ McGraw-Hill (www.digitalengineeringlibrary.com)
Copyright © 2004 The McGraw-Hill Companies. All rights reserved.
Any use is subject to the Terms of Use as given at the website.
Receiver Characteristics
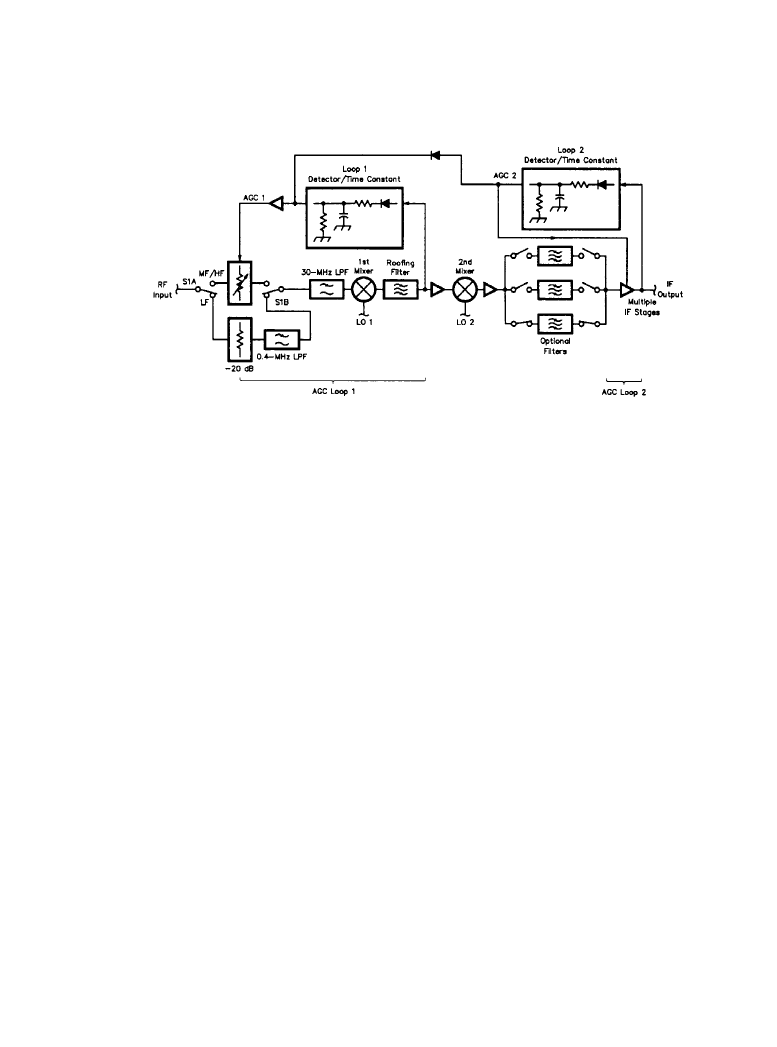
12-24 Broadcast Receiver Systems
The principal AGC characteristics of importance are the steady-state control range and out-
put-input curve, and the attack and decay times. In a some systems, a variety of time constants
are provided for the AGC to allow for different modulation types. For AM voice modulation, the
radiated carrier is constant and the lowest sidebands are usually several hundred hertz removed
from the carrier. At the receiver, the carrier component can be separated from the demodulated
wave by a low-pass filter and can serve as the AGC control voltage. The response time of the fil-
ter, which is often just an RC network, need only be fast enough to respond to the fading rate of
the medium, which is a maximum of 5 or 10 dB/s in most AM applications. A response time of
0.1 to 0.2 s is required for such a fading rate. For the more common slower rates, responses up to
a second or more can be used.
To test for the AGC range and input-output curve, a single signal generator is used (as in Fig-
ure 18.8.2) in the AM mode with the receiver’s AGC actuated. The signal generator is set to sev-
eral hundred microvolts, and the baseband output level is adjusted to a convenient level for
output power measurement. The signal generator is then tuned to its minimum level and the out-
put level is noted. The signal is gradually increased in amplitude, and the output level is mea-
sured for each input level, up to a maximum specified level, such as 2 V. Figure 12.1.12 shows
some typical AGC curves. In most cases, there will be a low-input region where the signal out-
put, rising out of the noise, varies linearly with the input. At some point, the output curve bends
over and begins to rise very slowly. At some high level, the output may drop off because of satu-
ration effects in some of the amplifiers. The point at which the linear relationship ends is the
AGC threshold of the AGC action. The point at which the output starts to decrease, if within a
specified range, is considered the upper end of the AGC control range. The difference between
these two input levels is the AGC control range. If the curve remains monotonic to the maximum
input test level, that level is considered the upper limit of the range. A measure of AGC effective-
ness is the increase in output from a specified lower input voltage level to an upper input voltage
Figure 12.1.11
Block diagram of a dual loop AGC system for a communications receiver.
Downloaded from Digital Engineering Library @ McGraw-Hill (www.digitalengineeringlibrary.com)
Copyright © 2004 The McGraw-Hill Companies. All rights reserved.
Any use is subject to the Terms of Use as given at the website.
Receiver Characteristics
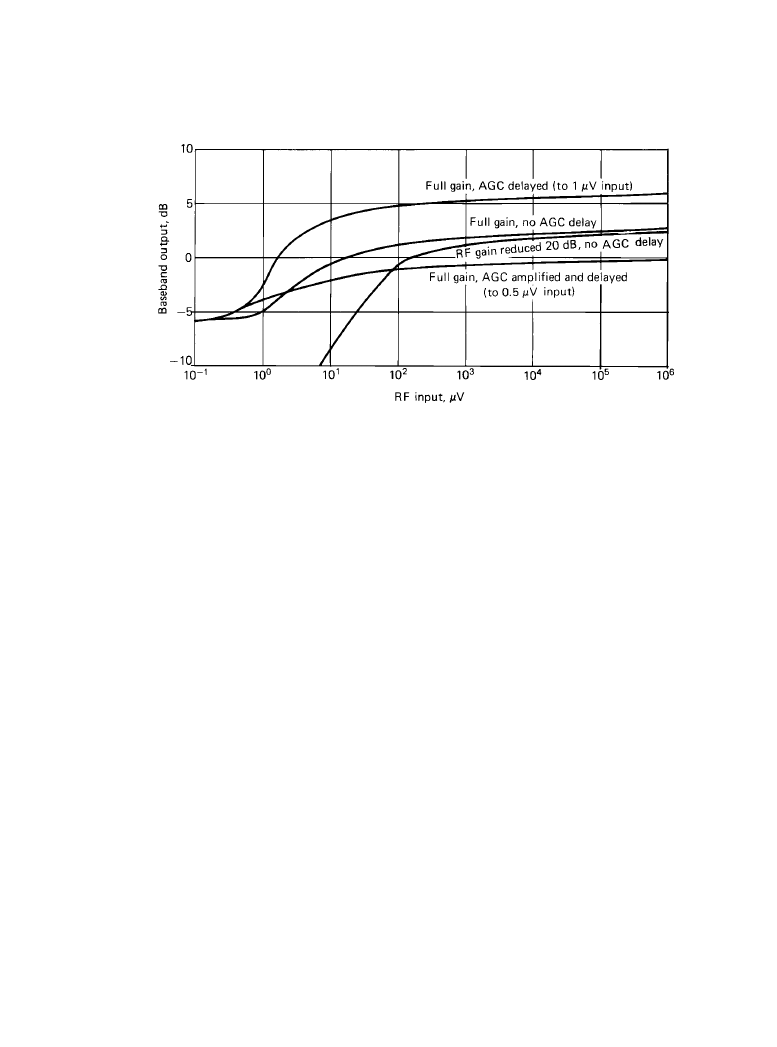
Receiver Characteristics 12-25
level. For example, a good design might have an AGC with a threshold below 1
µV that is mono-
tonic to a level of 1 V and has the 3 dB increase in output between 1
µV and 0.1 V.
The foregoing applies to a purely analog system. The advantage of a DSP-based system
include that AGC is handled internally. The receiver still requires a dual-loop AGC of which the
input stage AGC will remain analog.
12.1.6 Digital Receiver Characteristics
The foregoing has not exhausted the analog receiver characteristics that may be of interest but
has reviewed some of the more significant ones. For example, in FM sets, the capture ratio is
important. Clearly, an area of increasing interest is the characterization of systems utilizing digi-
tal modulation techniques. Because a digital radio system is a hybrid A/D device, many of the
test procedures outlined previously for analog receivers are useful and important in characteriz-
ing a digital radio system. Additional tests, primarily involving the analysis of bit error rates
(BER), must also be run to properly identify any weak points in a receiver design.
12.1.6a
BER Testing
The primary method for testing the quality of transmission over a high speed digital communica-
tions link is the BER, defined as the number of bit errors divided by the number of bits transmit-
ted. The BER is also used to qualify the sensitivity and noise characteristics of a receiver. The
major contributor to BER is jitter, which results from noise in the system. This noise causes the
output comparator to vary the time of its transition relative to the data clock. If the transition time
changes too much, an error will result
Figure 12.1.12
Representative input-output AGC curves.
Downloaded from Digital Engineering Library @ McGraw-Hill (www.digitalengineeringlibrary.com)
Copyright © 2004 The McGraw-Hill Companies. All rights reserved.
Any use is subject to the Terms of Use as given at the website.
Receiver Characteristics
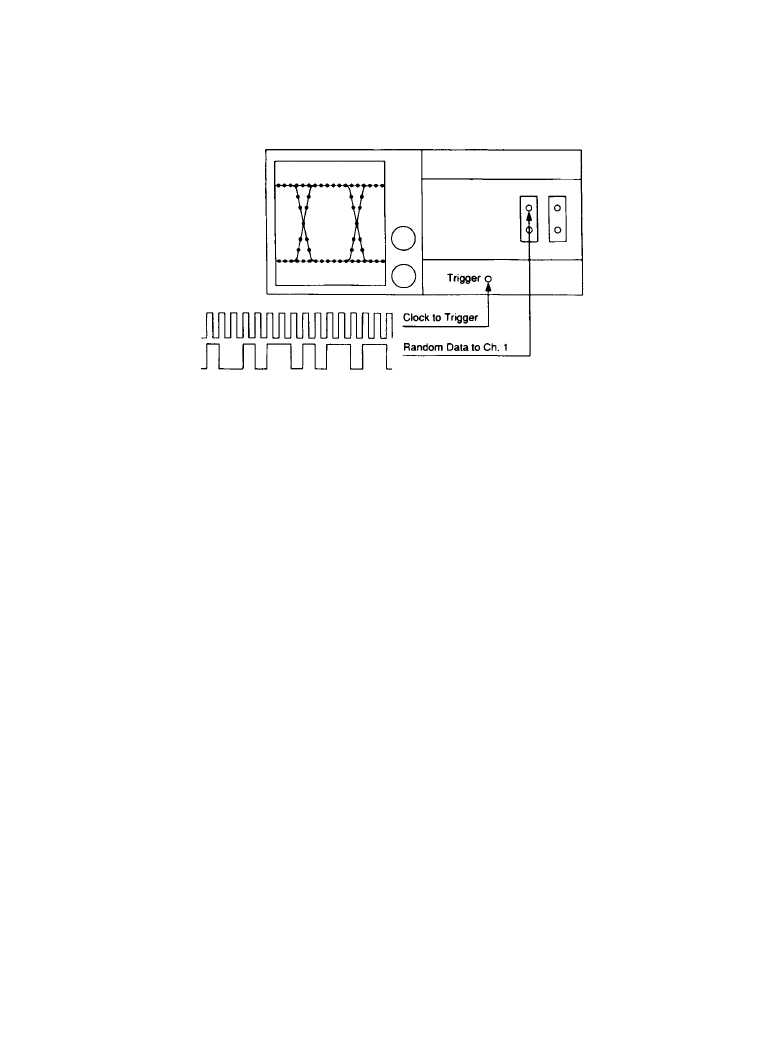
12-26 Broadcast Receiver Systems
Using a signal analyzer specifically designed for BER testing, jitter can be displayed directly,
or the BER is simply tabulated by the analyzer. The format of the testing signal is determined by
the application to which the digital radio system is applied. A variety of formats and protocols
are used. For end-to-end testing, the analyzer feeds a reference RF signal generator whose char-
acteristics are known, and the output signal is applied to the receiver antenna input.
The noise and jitter on a data waveform provides vital information about the quality of the
signal. A typical setup for capturing an eye pattern is shown in Figure 12.1.13. Eye patterns are
the traditional method of displaying high-speed digital data (Figure 12.1.14). Some communica-
tions signal analyzers augment this information with a built-in statistical database, which allows
additional analysis, including automated noise and jitter measurements on random data. Sophis-
ticated software can also analyze the form of the distribution, providing mean, rms, and standard
deviation results.
Proper receiver design involves identifying the areas of the system that are likely to cause
problems. LO phase noise is one such area. Phase noise can seriously impair the performance of
a digital receiver. Excessive phase noise can increase the BER, especially in systems using
phase-based modulation schemes, such as binary PSK and quadrature PSK. For a given statisti-
cal phase-error characteristic, BER is degraded according to the percentage of time that the
phase error causes the signal position in signal space to cross a decision boundary.
12.1.6b
Transmission and Reception Quality
Testing of digital circuits deviates from the typical analog measurements, and yet the analog
measurements are still necessary and related. In particular, because of the Doppler effect and the
use of digital rather than analog signals, where the phase information is significant, the designer
ends up using coding schemes for error-correction—specifically, forward error correction
(FEC). The S/N as we know it from analog circuits now determines the BER, and its tolerable
values depend on the type of modulation used. The actual bit error rate depends on the type of
filtering, coding, modulation, and demodulation.
Figure 12.1.13
Test setup for eye pattern measurement.
Downloaded from Digital Engineering Library @ McGraw-Hill (www.digitalengineeringlibrary.com)
Copyright © 2004 The McGraw-Hill Companies. All rights reserved.
Any use is subject to the Terms of Use as given at the website.
Receiver Characteristics
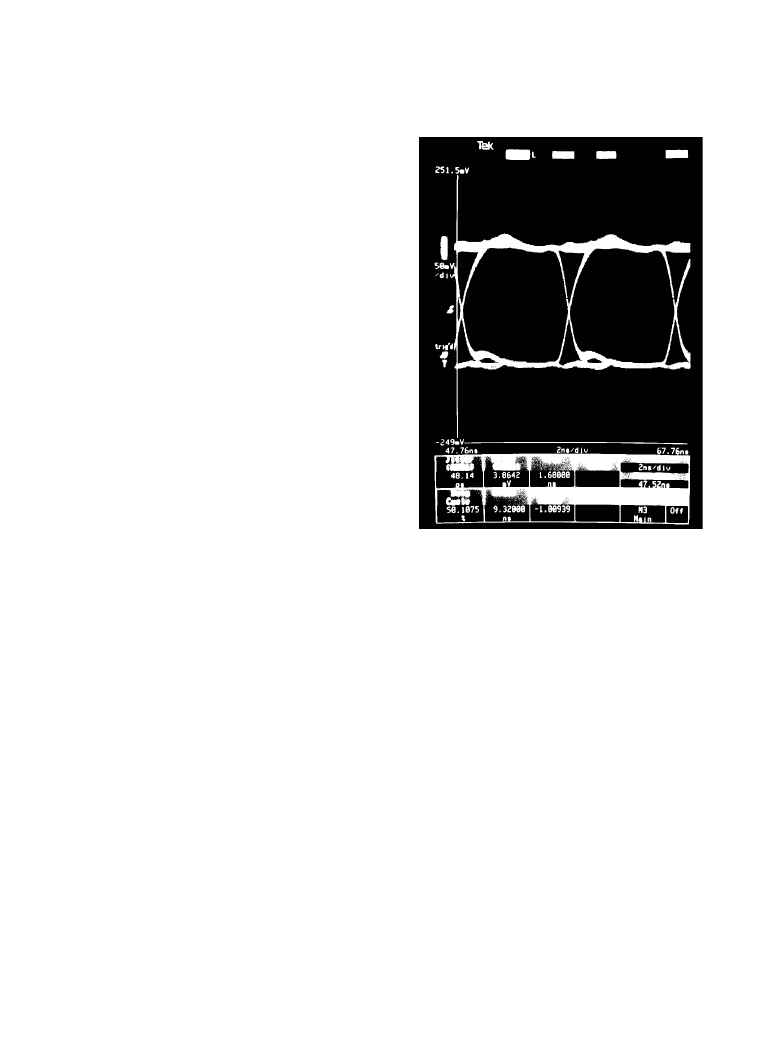
Receiver Characteristics 12-27
The adjacent-channel power ratio (ACPR), a factor involving the transmitter of a second sta-
tion, is another problem that receivers must deal with. Given the fact that a transmitter handling
digital modulation delivers its power in pulses, its transmissions may affect adjacent channels by
producing transient spurious signals similar to what we call splatter in analog SSB systems. This
is a function of the linearity of the transmitter system all the way out to the antenna, and forces
most designers to resort to less-efficient Class A operation. As possible alternatives, some
researchers have designed systems using Class D or E modulation.
It is not uncommon to do many linear measurements, and then by using correlation equations,
translate these measured results into their digital equivalents. Therefore, the robustness of the
signal as a function of antenna signal at the receiver site, constant or known phase relationships,
and high adjacent power ratios will provide good transfer characteristics.
12.1.7 Bibliography
Engelson, M., and J. Herbert: “Effective Characterization of CDMA Signals,” Microwave Jour-
nal, pg. 90, January 1995.
Howald, R.: “Understand the Mathematics of Phase Noise,” Microwaves & RF, pg. 97, Decem-
ber 1993.
Johnson, J. B:, “Thermal Agitation of Electricity in Conduction,” Phys. Rev., vol. 32, pg. 97, July
1928.
Figure 12.1.14
Eye pattern display of BER
measurement. (
Courtesy of Tektronix.)
Downloaded from Digital Engineering Library @ McGraw-Hill (www.digitalengineeringlibrary.com)
Copyright © 2004 The McGraw-Hill Companies. All rights reserved.
Any use is subject to the Terms of Use as given at the website.
Receiver Characteristics