ВУЗ: Казахская Национальная Академия Искусств им. Т. Жургенова
Категория: Книга
Дисциплина: Не указана
Добавлен: 03.02.2019
Просмотров: 21577
Скачиваний: 19
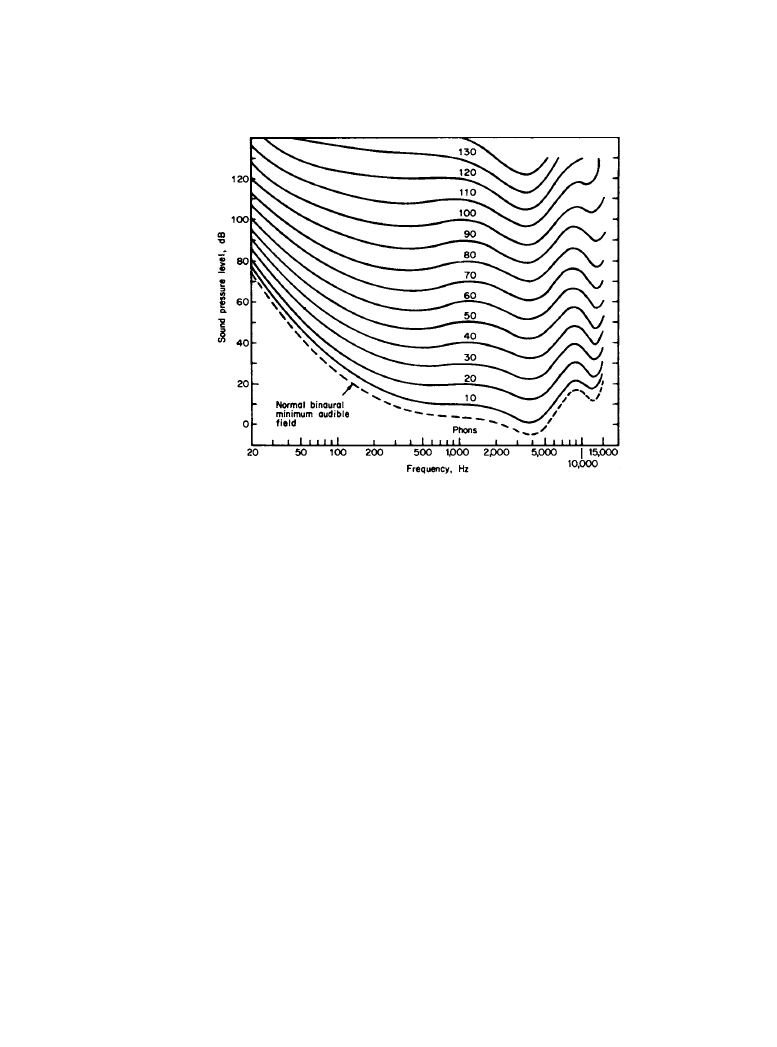
1-46 Principles of Sound and Hearing
pose of which is to boost progressively the bass frequencies as the overall sound level is reduced.
The design and use of such compensation have often been erroneous because of a confusion
between the shape of the loudness contours themselves and the differences between curves at
various phon levels [5]. Sounds reproduced at close to realistic levels should need no compensa-
tion, since the ears will respond to the sound just as they would to the “live” version of the pro-
gram. By the same token, control-room monitoring at very high sound levels can result in
program equalization that is note appropriate to reproduction at normal domestic sound levels
(combined with this are the effects of temporary and permanent changes in hearing performance
caused by exposure to loud sounds).
It is difficult to take the interpretations of equal-loudness contours much beyond generaliza-
tions since, as mentioned earlier, they are composites of data from many individuals. There is
also the fact that they deal with pure tones and the measurements were done either through head-
phones (Fletcher and Munson [2]) or in an anechoic chamber (Robinson and Dadson [3]). The
relationship between these laboratory tests and the common application for these data, the audi-
tion of music in normal rooms, is one that is only poorly established.
The lowest equal-loudness contour defines the lower limit of perception: the hearing-thresh-
old level. It is significant that the ears have their maximum sensitivity at frequencies that are
important to the intelligibility of speech. This optimization of the hearing process can be seen in
various other aspects of auditory performance as well.
The rate of growth of loudness as a function of the SPL is a matter of separate interest. Units
of sones are used to describe the magnitude of the subjective sensation. One sone is defined as
Figure 1.4.2
Contours of equal loudness showing the sound pressure level required for pure tones
at different frequencies to sound as loud as a reference tone of 1000 Hz. (
From ISO Recommen-
dation R226.)
Downloaded from Digital Engineering Library @ McGraw-Hill (www.digitalengineeringlibrary.com)
Copyright © 2004 The McGraw-Hill Companies. All rights reserved.
Any use is subject to the Terms of Use as given at the website.
The Physical Nature of Hearing
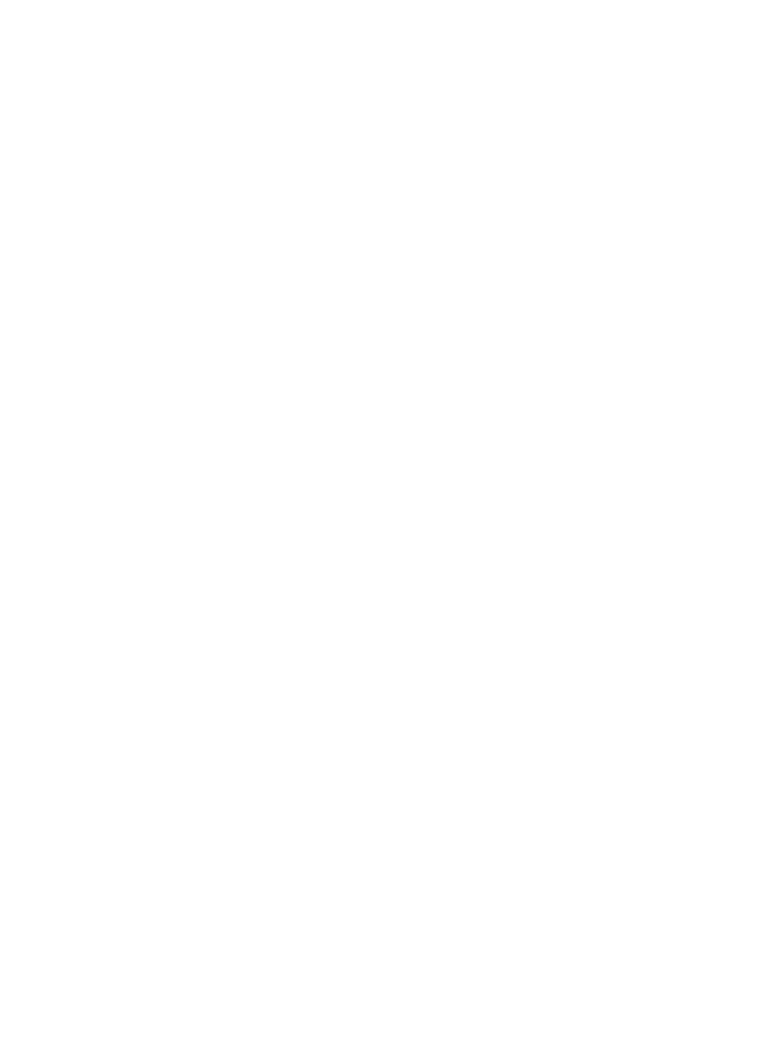
The Physical Nature of Hearing 1-47
the loudness of a tone at the 40-phon loudness level. A sound of loudness 2 sones would be twice
as loud, and a sound of 0.5 sone would be half as loud. The loudness function relating the subjec-
tive sensation to the physical magnitude has been studied extensively [6], and while there are
consistencies in general behavior, there remain very large differences in the performance of indi-
viduals and in the effect of the temporal and spectral structure of the sound. A common approxi-
mation relates a change of 10 dB in SPL to a doubling or halving of loudness. Individual
variations on this may be a factor of 2 or more, indicating that one is not dealing with precise
data. For example, the growth of loudness ate low frequencies, as shown in the curves of Figure
1.4.2, indicates a clear departure from the general rule. Nevertheless, it is worth noting that sig-
nificant changes in loudness require large differences in SPL and sound power; a doubling of
loudness that requires a l0-dB increase in sound level translates into a factor of 3.16 in sound
pressure (or voltage) and a factor of 10 in power.
Loudness as a Function of Bandwidth
The studies of loudness that used pure tones leave doubts about how they relate to normal sounds
that are complexes of several frequencies or continuous bands of sound extending over a range of
frequencies. If the bandwidth of a sound is increased progressively while maintaining a constant
overall measured sound level, it is found that loudness remains constant from narrow bandwidths
up to a value called the critical bandwidth. At larger bandwidths, the loudness increases as a
function of bandwidth because of a process known as loudness summation. For example, the
broadband sound of an orchestra playing a chord will be louder than the simple sound of a flute
playing a single note even when the sounds have been adjusted to the same SPL.
The critical bandwidth varies with the center frequency of the complex sound being judged.
At frequencies below about 200 Hz it is fairly constant at about 90 Hz; at higher frequencies the
critical bandwidth increases progressively to close to 4000 Hz at 15 kHz. The sound of the
orchestra therefore occupies many critical bandwidths while the sound of the flute is predomi-
nantly within one band.
Loudness as a Function of Duration
Brief sounds can appear to be less loud than sounds with the same maximum sound level but
longer duration. Experiments show that there is a progressive growth of loudness as signal dura-
tion is increased up to about 200 ms; above that, the relationship levels out. The implication is
that the hearing system integrates sound energy over a time interval of about 200 ms. In reality,
the integration is likely to be of neural energy rather than acoustical energy, which makes the pro-
cess rather complicated, since it must embrace all the nonlinearities of the perceptual mecha-
nism.
The practical consequence of this is that numerous temporal factors, such as duration, inter-
mittency, repetition rate, and so on, all influence the loudness of sounds that are separate from
SPL.
Measuring the Loudness of Complex Sounds
Given the numerous variables and uncertainties in ascertaining the loudness of simple sounds, it
should come as no surprise that measuring the loudness of the wideband, complex, and ever-
changing sounds of real life is a problem that has resisted simple interpretation. Motivated by the
need to evaluate the annoyance value of sounds as well as the more neutral quantity of loudness,
Downloaded from Digital Engineering Library @ McGraw-Hill (www.digitalengineeringlibrary.com)
Copyright © 2004 The McGraw-Hill Companies. All rights reserved.
Any use is subject to the Terms of Use as given at the website.
The Physical Nature of Hearing
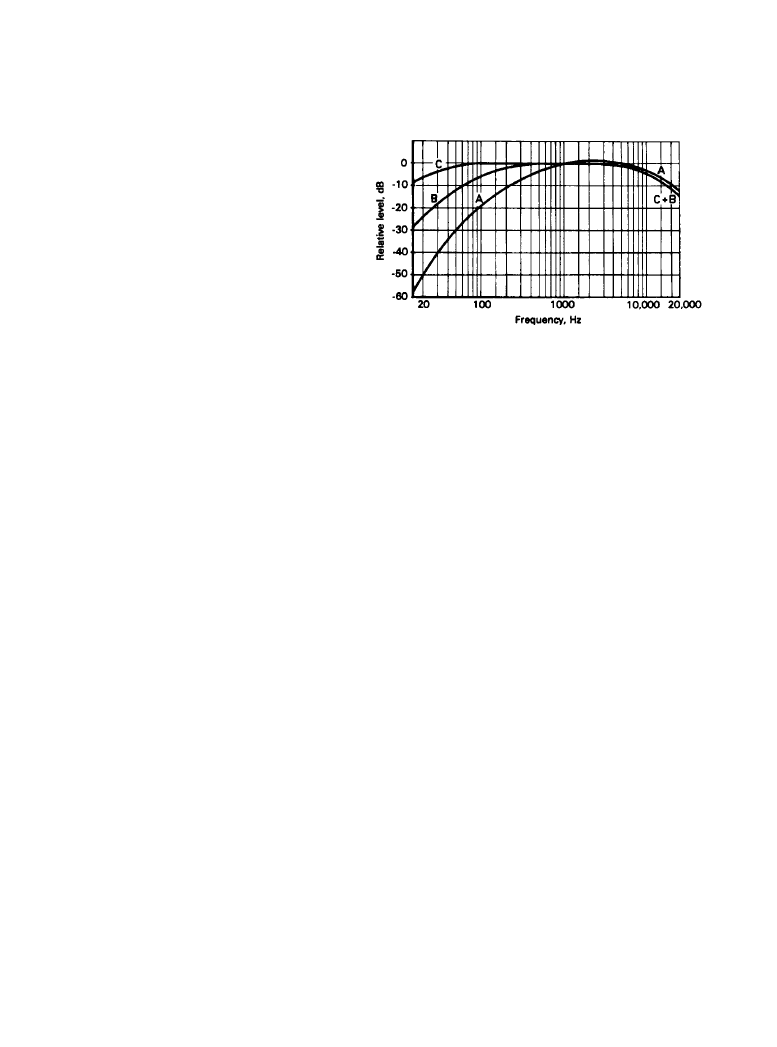
1-48 Principles of Sound and Hearing
various methods have been developed for arriving at single-number ratings of complex sounds.
Some methods make use of spectral analysis of the sound, adjusted by correction factors and
weighting, to compute a single-number loudness rating. These tend to require expensive appara-
tus and are, at best, cumbersome to use; they also are most accurate for steady-state sounds.
Simplifying the loudness compensation permits the process to be accomplished with rela-
tively straightforward electronics providing a direct-reading output in real time, a feature that
makes the device practical for recording and broadcasting applications. Such devices are
reported to give rather better indications of the loudness of typical music and speech program
material than the very common and even simpler volume-unit (VU) meters or sound-level meters
[7].
The VU meter responds to the full audio-frequency range, with a flat frequency response but
with some control of its dynamic (time) response. A properly constructed VU meter should
exhibit a response time of close to 300 ms, with an overswing of not more than 1.5 percent, and a
return time similar to the response time. The dial calibrations and reference levels are also stan-
dardized. Such devices are therefore useful for measuring the magnitudes of steady-state signals
and for giving a rough indication of the loudness of complex and time-varying signals, but they
fail completely to take into account the frequency dependence of loudness.
The sound-level meters used for acoustical measurements are adjustable in both amplitude
and time response. Various frequency-weighting curves, A-weighting being the most popular,
acknowledge the frequency-dependent aspects of loudness, and “fast” and “slow” time responses
deal differently with temporal considerations. Although these instruments are carefully standard-
ized and find extensive use in acoustics, noise control, and hearing conservation, they are of lim-
ited use as program-level indicators. Figure 1.4.3 shows the common frequency-weighting
options found in sound-level meters. A-weighting has become the almost universal choice for
measurements associated with loudness, annoyance, and the assessment of hearing-damage risk.
Peak program meters (PPM) are also standardized [8], and they find extensive use in the
recording and broadcast industries. However, they are used mainly as a means of avoiding over-
loading recorders and signal-processing equipment. Consequently, the PPM has a very rapid
response (an integration time of about 10 ms in the normal mode), so that brief signal peaks are
registered, and a slow return (around 3 s), so that the peak levels can be easily seen. These
devices therefore are not useful indicators of loudness of fluctuating signals.
Figure 1.4.3
The standard fre-
quency-weighting networks used in
sound-level meters.
Downloaded from Digital Engineering Library @ McGraw-Hill (www.digitalengineeringlibrary.com)
Copyright © 2004 The McGraw-Hill Companies. All rights reserved.
Any use is subject to the Terms of Use as given at the website.
The Physical Nature of Hearing
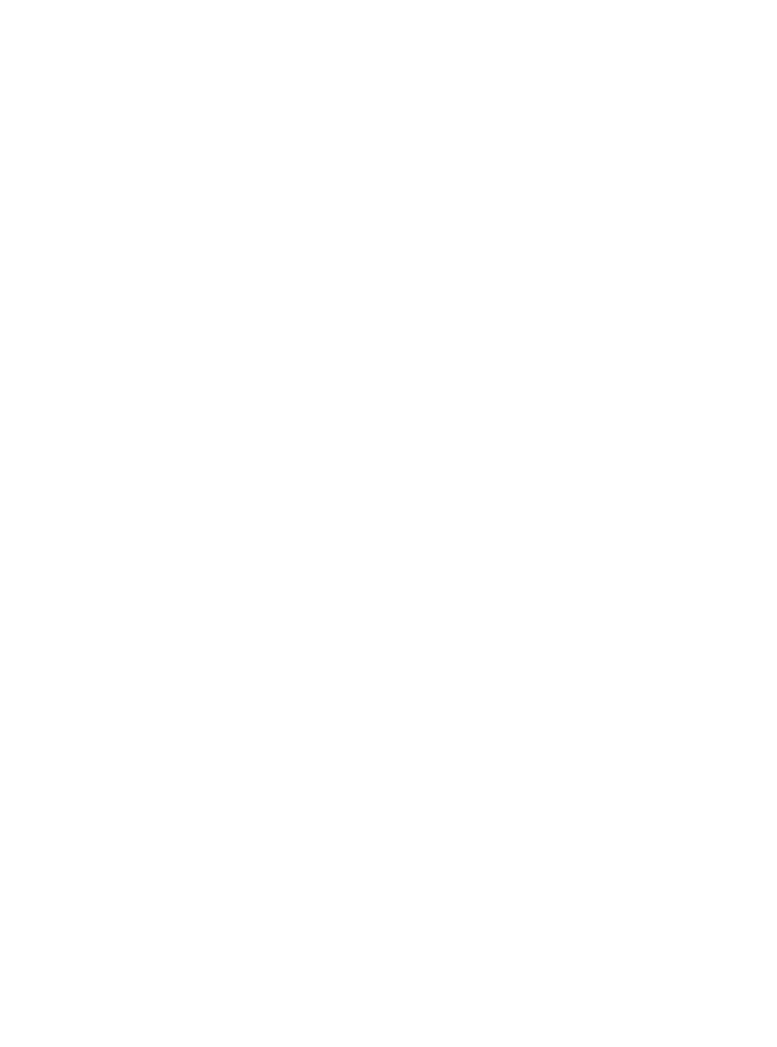
The Physical Nature of Hearing 1-49
1.4.3b
Masking
Listening to a sound in the presence of another sound, which for the sake of simplicity we shall
call noise, results in the desired sound being, to some extent, less audible. This effect is called
masking. If the noise is sufficiently loud, the signal can be completely masked, rendering it inau-
dible; at lower noise levels the signal will be partially masked, and only its apparent loudness
may be reduced. If the desired sound is complex, it is possible for masking to affect only portions
of the total sound. All this is dependent on the specific nature of both the signal and the masking
sound.
In audio it is possible for the low-level sounds of music, for example, to be masked by back-
ground noise in a sound system. That same noise can mask distortion products, so the effects
need not be entirely undesirable. In addition to the unwanted noises that have been implied so far,
there can be masking of musical sounds by other musical sounds. Thus we encounter the interest-
ing situation of the perceived sound of a single musical instrument modified by the sounds of
other instruments when it is joined in an ensemble.
In addition to the partial and complete masking that occurs when two sounds occur simulta-
neously, there are instances of temporal masking, when the audibility of a sound is modified by a
sound that precedes it in time (forward masking) or, strange as it may seem, by a sound that fol-
lows it (backward masking).
Simultaneous Masking
At the lowest level of audibility, the threshold, the presence of noise can cause a threshold shift
wherein the amplitude of the signal must be increased to restore audibility. At higher sound lev-
els the masked sound may remain audible but, owing to partial masking, its loudness can be
reduced.
In simultaneous masking the signal and the masking sound coexist in the time domain. It is
often assumed that they must also share the same frequency band. While this seems to be most
effective, it is not absolutely necessary. The effect of a masking sound can extend to frequencies
that are both higher and lower than those in the masking itself At low sound levels a masking
sound tends to influence signals with frequencies close to its own, but at higher sound levels the
masking effect spreads to include frequencies well outside the spectrum of the masker. The dom-
inant effect is an upward spread of masking that can extend several octaves above the frequency
of the masking sound. There is also a downward spread of masking, but the effect is considerably
less. In other words, a low-frequency masking sound can reduce the audibility of higher-fre-
quency signals, but a high-frequency masking sound has relatively little effect on signals of
lower frequency. Figure 1.4.4 shows that a simple masking sound elevates the hearing threshold
over a wide frequency range but that the elevation is greater for frequencies above the masking
sound.
In the context of audio, this means that we have built-in noise and distortion suppression.
Background noises of all kinds are less audible while the music is playing but stand out clearly
during the quiet intervals. Distortions generated in the recording and reproduction processes are
present only during the musical sound and are therefore at least partially masked by the music
itself This is especially true for harmonic distortions, in which the objectionable distortion prod-
ucts are at frequencies higher than the masking sound—the sound that causes them to exist.
Intermodulation-distortion products, on the other hand, are at frequencies both above and below
Downloaded from Digital Engineering Library @ McGraw-Hill (www.digitalengineeringlibrary.com)
Copyright © 2004 The McGraw-Hill Companies. All rights reserved.
Any use is subject to the Terms of Use as given at the website.
The Physical Nature of Hearing
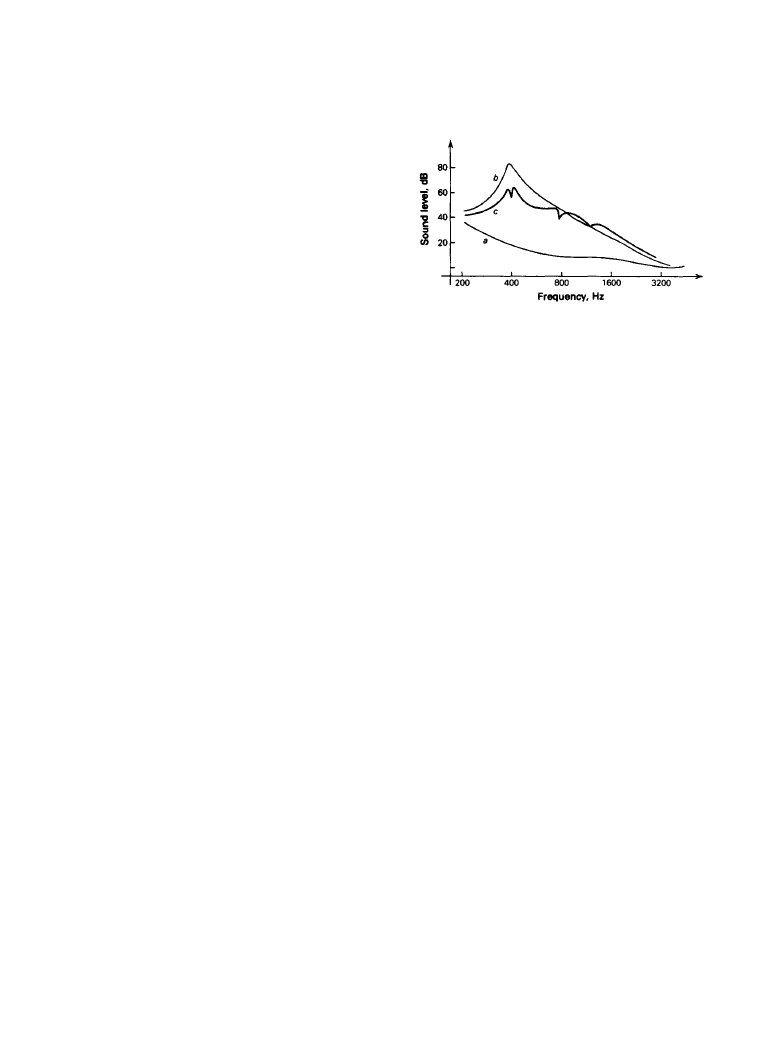
1-50 Principles of Sound and Hearing
the frequencies of the signals that produce the distortion. In this case, the upper distortion prod-
ucts will be subject to greater masking by the signal than the lower distortion products.
Studies of distortion have consistently noted that all forms of distortion are less audible with
music than with simple signals such as single tones or combinations of tones; the more effective
masking of the spectrally complex music signal is clearly a factor in this. Also noted is that inter-
modulation distortion is more objectionable than its harmonic equivalent. A simple explanation
for this may be that not only are the difference-frequency components of intermodulation distor-
tion unmusical, but they are not well masked by the signals that produce them.
Temporal Masking
The masking that occurs between signals not occurring simultaneously is known as temporal
masking. It can operate both ways, from an earlier to a later sound (forward masking) or from a
later to an earlier sound (backward masking). The apparent impossibility of backward masking
(going backward in time) has a physiological explanation. It takes time for sounds to be pro-
cessed in the peripheral auditory system and for the neural information to travel to the brain. If
the later sound is substantially more intense than the earlier sound, information about it can take
precedence over information about the earlier sound. The effect can extend up to 100 to 200 ms,
but because such occurrences are rare in normal hearing, the most noteworthy auditory experi-
ences are related to forward masking.
Forward masking results from effects of a sound that remain after the physical stimulus has
been removed. The masking increases with the sound level of the masker and diminishes rapidly
with time, although effects can sometimes be seen for up to 500 ms [9]. Threshold shifts of 10 to
20 dB appear to be typical for moderate sound levels, but at high levels these may reach 40 to 50
dB. Combined with these substantial effects is a broadening of the frequency range of the mask-
ing; at masker sound levels above about 80 dB maximum masking no longer occurs at the fre-
quency of the masking sound but at higher frequencies.
There are complex interactions among the numerous variables in the masking process, and it
is difficult to translate the experimental findings into factors specifically related to audio engi-
neering. The effects are not subtle, however, and it is clear that in many ways they influence what
we hear.
Figure 1.4.4
Detection threshold for pure
tones of various frequencies: (
a) in insola-
tion, (
b) in the presence of a narrow band
(365 to 455 Hz) of masking noise centered
on 400 Hz at a sound level of 80 dB, (
c) in
the presence of a making tone of 400 Hz at
80 dB. (
From [40]. Used with permission.)
Downloaded from Digital Engineering Library @ McGraw-Hill (www.digitalengineeringlibrary.com)
Copyright © 2004 The McGraw-Hill Companies. All rights reserved.
Any use is subject to the Terms of Use as given at the website.
The Physical Nature of Hearing