ВУЗ: Казахская Национальная Академия Искусств им. Т. Жургенова
Категория: Книга
Дисциплина: Не указана
Добавлен: 03.02.2019
Просмотров: 21573
Скачиваний: 19
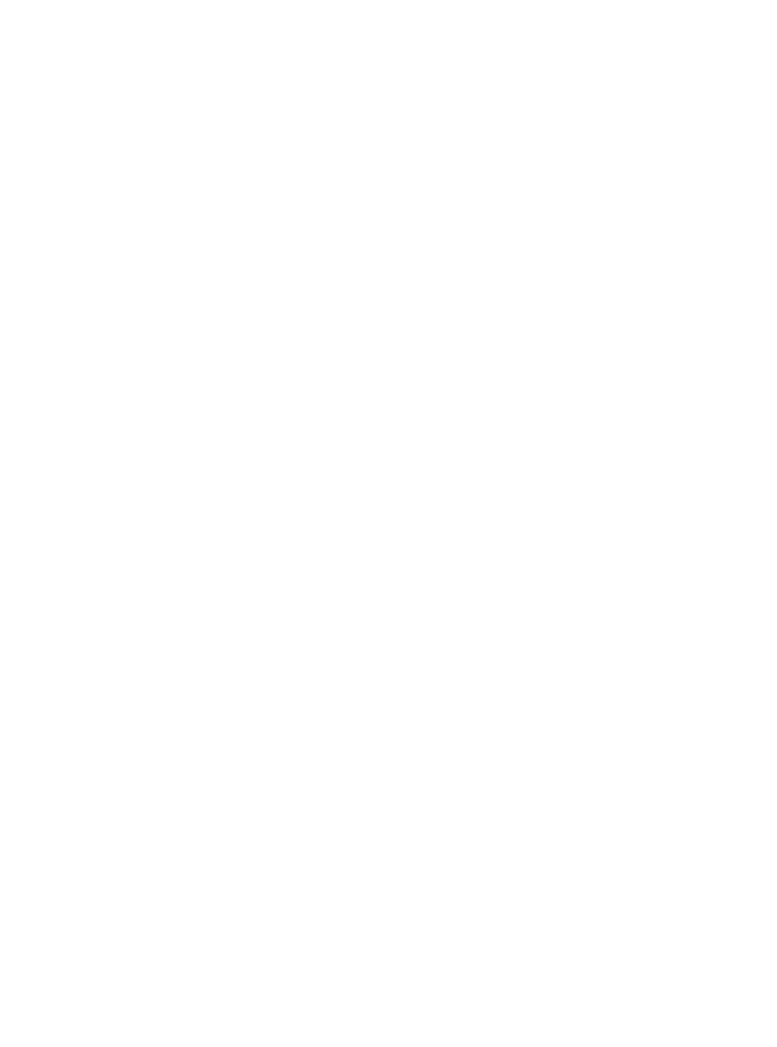
Resonance 1-41
ing in closely predictable performance at low frequencies. At higher frequencies, standing waves
form inside the box, and the tidy lumped-element concepts no longer apply.
1.3.2d
Horns
If the open end of a tube has a diameter that is small compared with the wavelength of sound
being propagated within it, most of the sound is reflected back into the tube, and if the wave-
length is appropriate, standing waves result. At resonance, the acoustical activity is at its maxi-
mum, but the small tube opening is nevertheless a rather inefficient radiator of sound. If strong
resonances are important and adequate input power is available, as in organ pipes, this is a desir-
able situation. Other devices, however, require the maintenance of strong standing waves, but
with an improved radiation efficiency. With care this is achieved through the use of a flared
development, or horn, at the end of the pipe. The shape and size of the horn determine, for every
frequency, how much of the sound is reflected back into the tube and how much radiates out-
ward.
The musical instruments of the brass family are all combinations of resonant pipes with a
flaring bell at the output end. The shape of a trumpet bell, for example, is such that it has radia-
tion efficiency that is low below about 1500 Hz and high above. By establishing strong reso-
nances at the fundamental playing frequencies, the bell makes the instrument playable while
imparting a bright sound character by efficiently radiating the higher harmonics of the basic
pitch [7, 8].
On the other hand, a loudspeaker horn must have high radiation efficiency at all frequencies
within its operating range; otherwise there will be resonances in a system that is intended to be
free of such sources of tone color. The key to non-resonant behavior lies in the choice of flare
shape and mouth size. The sound waves propagating outward must be allowed to expand at just
the proper rate, maintaining close control over the directions of the particle velocities, so that the
waves can emerge from the enlarged mouth with little energy reflected back to the loudspeaker.
[5].
1.3.3
References
1.
Main, Ian G.: Vibrations and Waves in Physics, Cambridge, London, 1978.
2.
Pickett, J. M.: The Sounds of Speech Communication, University Park Press, Baltimore,
MD, 1980.
3.
Denes, Peter B., and E. N. Pinson: The Speech Chain, Bell Telephone Laboratories,
Waverly, 1963.
4.
Sundberg, Johan: “The Acoustics of the Singing Voice,” in The Physics of Music, introduc-
tion by C. M. Hutchins, Scientific American/Freeman, San Francisco, Calif., 1978.
5.
Morse, Philip M.: Vibrations and Sound, 1964, reprinted by the Acoustical Society of
America, New York, N.Y., 1976.
6.
Mankovsky, V. S.: Acoustics of Studios and Auditoria, Focal Press, London, 1971.
7.
Hall, Donald E.: Musical Acoustics: An Introduction, Wadsworth, Belmont, Calif, 1980.
Downloaded from Digital Engineering Library @ McGraw-Hill (www.digitalengineeringlibrary.com)
Copyright © 2004 The McGraw-Hill Companies. All rights reserved.
Any use is subject to the Terms of Use as given at the website.
Resonance
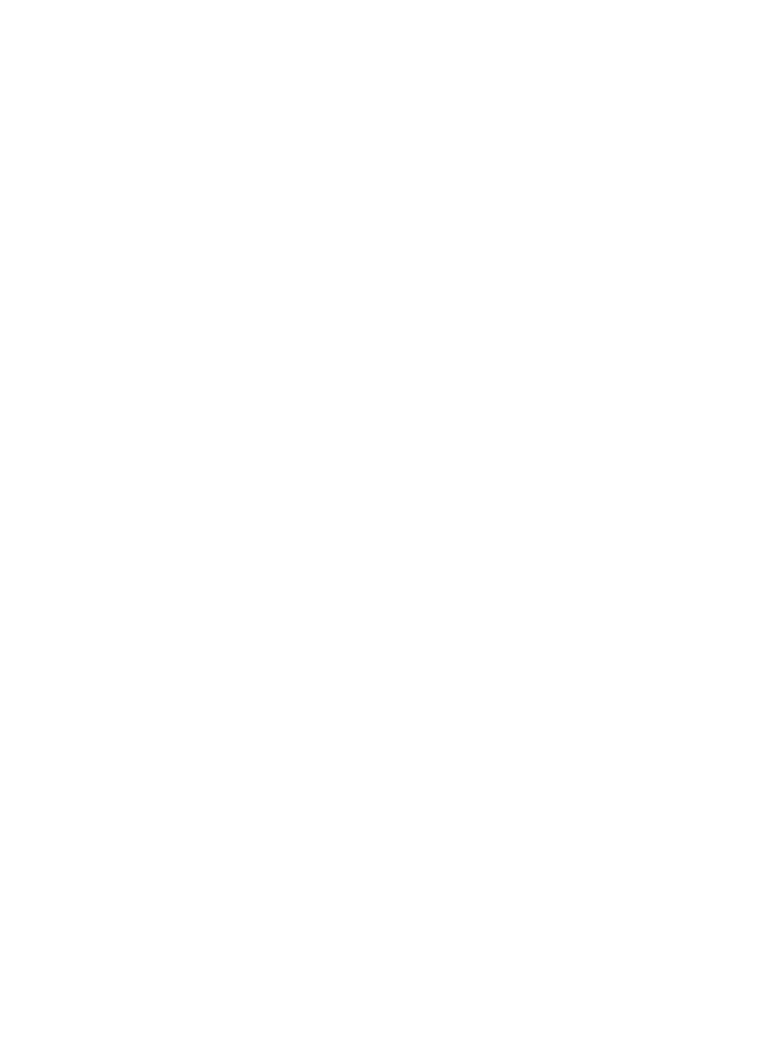
1-42 Principles of Sound and Hearing
8.
Benade, A. H.: Fundamentals of Musical Acoustics, Oxford University Press, New York,
N.Y., 1976.
Downloaded from Digital Engineering Library @ McGraw-Hill (www.digitalengineeringlibrary.com)
Copyright © 2004 The McGraw-Hill Companies. All rights reserved.
Any use is subject to the Terms of Use as given at the website.
Resonance
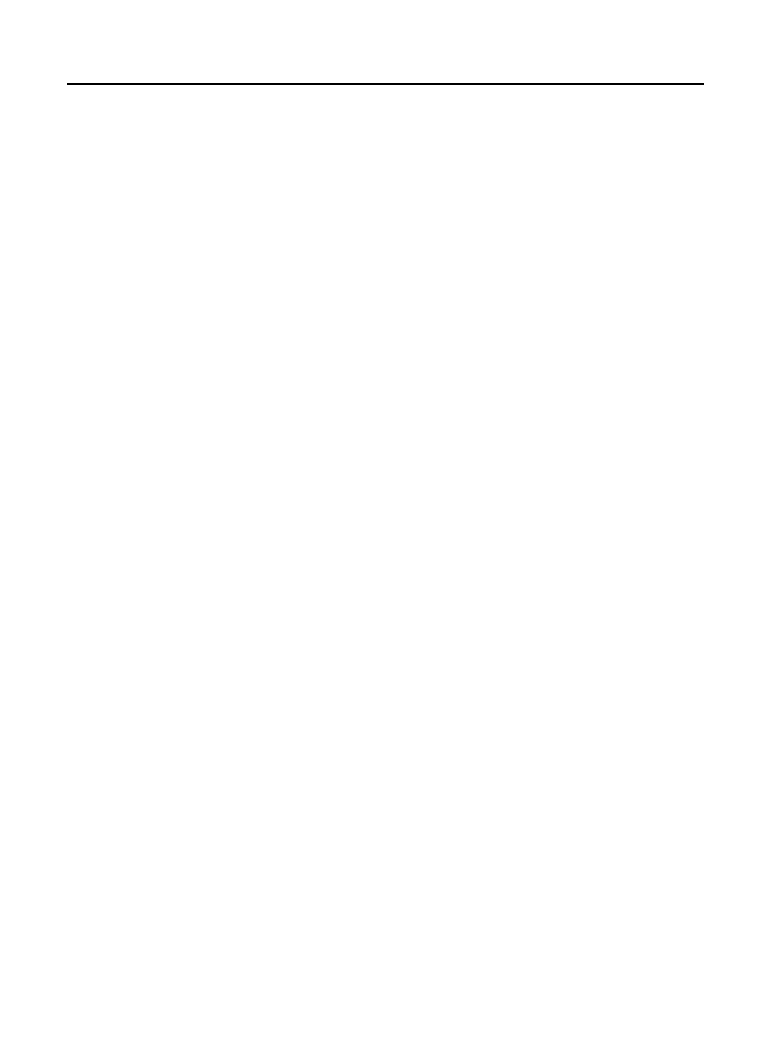
1-43
Chapter
1.4
The Physical Nature of Hearing
Floyd E. Toole
E. A. G. Shaw, G. A. Daigle, M. R. Stinson
1.4.1
Introduction
The process of hearing begins with acoustical modifications to the sound waves as they interact
with the head and the external ear, the visible portion of the system. These acoustical changes are
followed by others in the ear canal and by a conversion of the sound pressure fluctuations into
mechanical displacements by the eardrum. Transmitted through the mechanical coupling system
of the middle ear to the inner ear, the displacement patterns are partially analyzed and then
encoded in the form of neural signals. The signals from the two ears are cross-compared at sev-
eral stages on the way to the auditory centers of the brain, where finally there is a transformation
of the streams of data into perceptions of sound and acoustical space.
By these elaborate means we are able to render intelligible acoustical signals that, in technical
terms, can be almost beyond description. In addition to the basic information, the hearing pro-
cess keeps us constantly aware of spatial dimensions, where sounds are coming from, and the
general size, shape, and decor of the space around us—a remarkable process indeed.
1.4.2
Anatomy of the Ear
Figure l.4.1a shows a cross section of the ear in a very simplified form in which the outer, mid-
dle, and inner ear are clearly identified. The head and the outer ear interact with the sound waves,
providing acoustical amplification that is dependent on both direction and frequency, in much
the same way as an antenna. At frequencies above about 2 kHz there are reflections and reso-
nances in the complex folds of the pinna [1]. Consequently, sounds of some frequencies reach
the tympanic membrane (eardrum) with greater amplitude than sounds of other frequencies. The
amount of the sound pressure gain or loss depends on both the frequency and the angle of inci-
dence of the incoming sound. Thus, the external ear is an important first step in the perceptual
process, encoding sounds arriving from different directions with distinctive spectral characters.
For example, the primary resonance of the external ear, at about 2.6 kHz, is most sensitive to
Downloaded from Digital Engineering Library @ McGraw-Hill (www.digitalengineeringlibrary.com)
Copyright © 2004 The McGraw-Hill Companies. All rights reserved.
Any use is subject to the Terms of Use as given at the website.
Source: Standard Handbook of Audio and Radio Engineering
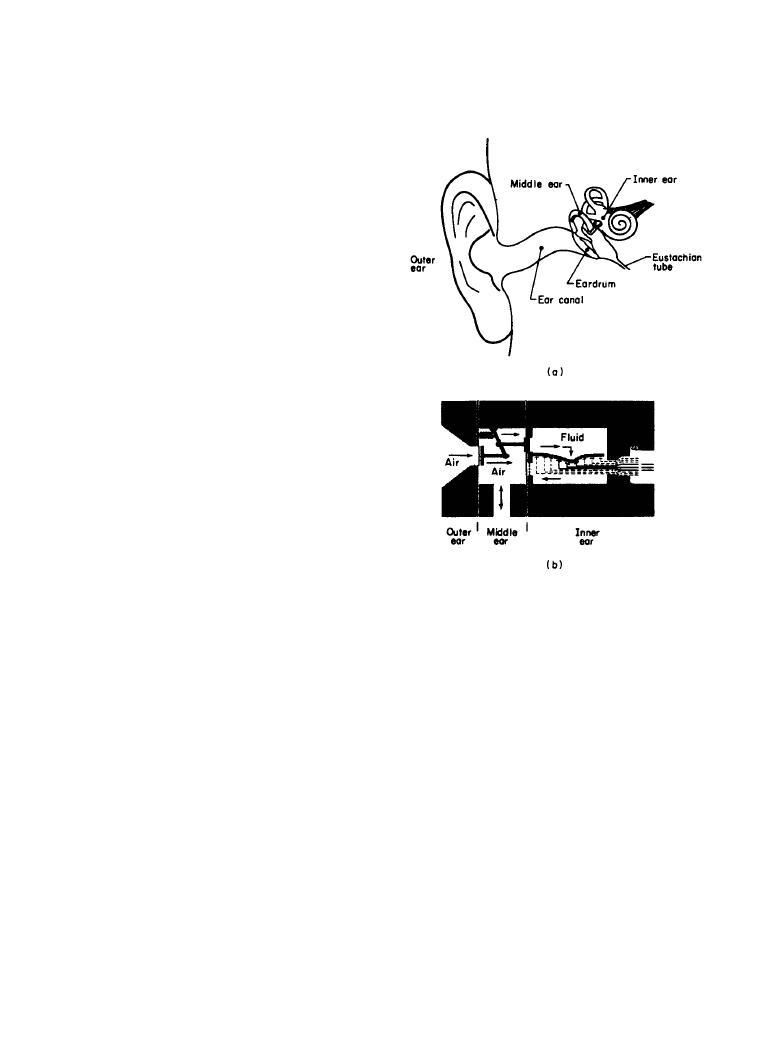
1-44 Principles of Sound and Hearing
sounds arriving from near 45° azimuth. This can be demonstrated by listening to a source of
broadband sound while looking directly at it and then slowly rotating the head until one ear is
pointing toward it. As the head is rotated through 45°, the sound should take on a “brighter”
character as sounds in the upper midrange are accentuated. People with hearing problems use
this feature of the ear to improve the intelligibility of speech when they unconsciously tilt the
head, directing the ear toward the speaker. Continuing the rotation reveals a rapid dulling of the
sound as the source moves behind the head. This is caused by acoustical shadowing due to dif-
fraction by the pinna, a feature that helps to distinguish between front and back in sound localiza-
tion.
At the eardrum the sound pressure fluctuations are transformed into movement that is coupled
by means of the middle-ear bones (the ossicular chain) to the oval window, the input to the inner
ear (cochlea). The middle ear increases the efficiency of sound energy transfer by providing a
partial impedance match between sound in air, on the one hand, and wave motion in the liquid-
filled inner ear, on the other. The inner ear performs the elaborate function of analyzing the
sound into its constituent frequencies and converting the result into neural signals that pass up
the auditory (eighth) nerve to the auditory cortex of the brain. From there sound is transformed
into the many and varied perceptions that we take for granted. In the following discussions we
shall be dealing with some of these functions in more detail.
Figure 1.4.1
The human ear: (
a) cross-sec-
tional view showing the major anatomical
elements, (
b) a simplified functional repre-
sentation.
Downloaded from Digital Engineering Library @ McGraw-Hill (www.digitalengineeringlibrary.com)
Copyright © 2004 The McGraw-Hill Companies. All rights reserved.
Any use is subject to the Terms of Use as given at the website.
The Physical Nature of Hearing
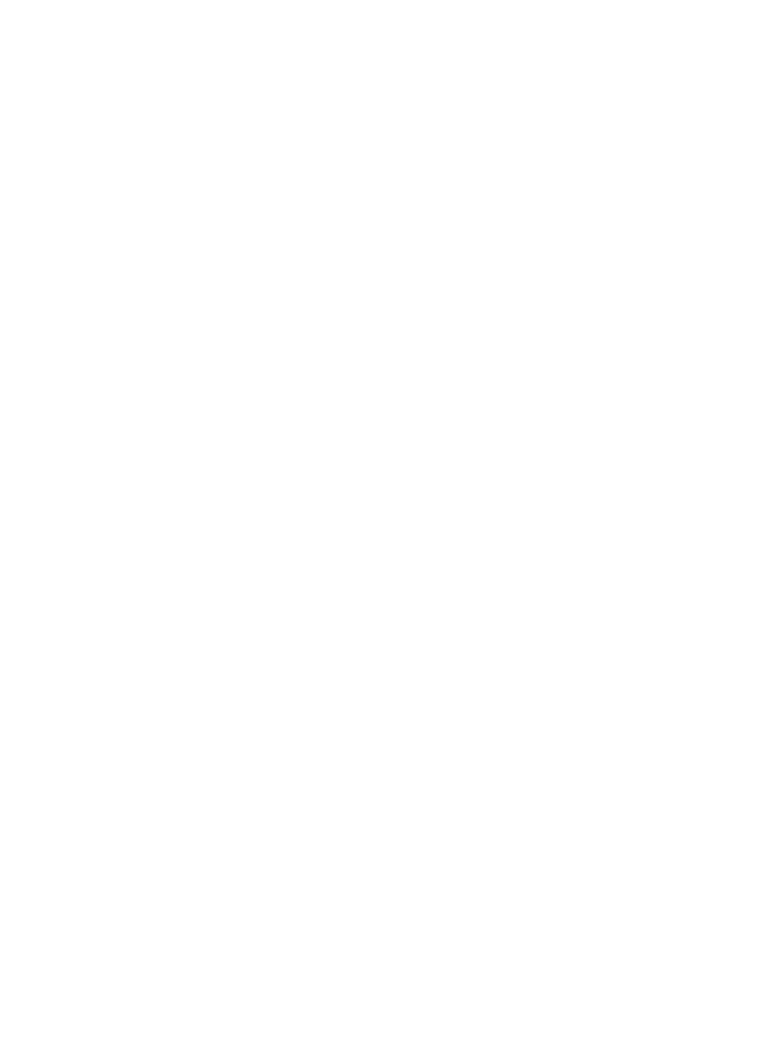
The Physical Nature of Hearing 1-45
1.4.3
Psychoacoustics and the Dimensions of Hearing
The physical dimensions of sound have parallels in the perceptual processes. The relationships
are usually nonlinear, more complex than at first appearance, and somewhat variable among
individuals as well as with time and experience. Nevertheless, they are the very essence of hear-
ing.
The study of these relationships falls under the general umbrella of psycho-acoustics. A more
specialized study, known as psychophysics or psychometrics, is concerned with quantification of
the magnitudes of the sensation in relation to the magnitude of the corresponding physical stim-
ulus.
1.4.3a
Loudness
Loudness is the term used to describe the magnitude of an auditory sensation. It is primarily
dependent upon the physical magnitude (sound pressure) of the sound producing the sensation,
but many other factors are influential.
Sounds come in an infinite variety of frequencies, timbres, intensities, temporal patterns, and
durations; each of these, as well as the characteristics of the individual listener and the context
within which the sound is heard, has an influence on loudness. Consequently, it is impossible for
a single graph or equation to accurately express the relationship between the physical quality and
quantity of sound and the subjective impression of loudness. Our present knowledge of the phe-
nomenon is incomplete, but there are some important experimentally determined relationships
between loudness and certain measurable quantities of sound. Although it is common to present
and discuss these relationships as matters of fact, it must always be remembered that they have
been arrived at through the process of averaging the results of many experiments with many lis-
teners. These are not precise engineering data; they are merely indicators of trends.
Loudness as a Function of Frequency and Amplitude
The relationship between loudness and the frequency and SPL of the simplest of sounds, the pure
tone, was first established by Fletcher and Munson, in 1933 [2]. There have been several subse-
quent redeterminations of loudness relationships by experimenters incorporating various refine-
ments in their techniques. The data of Robinson and Dadson [3], for example, provide the basis
for the International Organization for Standardization (ISO) recommendation R226 [4]. The pre-
sentation of loudness data is usually in the form of equal-loudness contours, as shown in Figure
1.4.2. Each curve shows the SPLs at which tones of various frequencies are judged to sound
equal in loudness to a l-kHz reference tone; the SPL of the reference tone identifies the curve in
units called phons. According to this method, the loudness level of a sound, in phons, is the SPL
level of a l-kHz pure tone that is judged to be equally loud.
The equal-loudness contours of Figure 1.4.2 show that the ears are less sensitive to low fre-
quencies than to middle and high frequencies and that this effect increases as sound level is
reduced. In other words, as the overall sound level of a broadband signal such as music is
reduced, the bass frequencies will fade faster than middle or high frequencies. In the curves, this
appears as a crowding together of the contours at low frequencies, indicating that, at the lower
sound levels, a small change in SPL of low-frequency sounds produces the same change in loud-
ness as a larger change in SPL at middle and high frequencies. This may be recognized as the
basis for the loudness compensation controls built into many domestic hi-fi amplifiers, the pur-
Downloaded from Digital Engineering Library @ McGraw-Hill (www.digitalengineeringlibrary.com)
Copyright © 2004 The McGraw-Hill Companies. All rights reserved.
Any use is subject to the Terms of Use as given at the website.
The Physical Nature of Hearing