ВУЗ: Казахская Национальная Академия Искусств им. Т. Жургенова
Категория: Учебное пособие
Дисциплина: Не указана
Добавлен: 03.02.2019
Просмотров: 17353
Скачиваний: 18
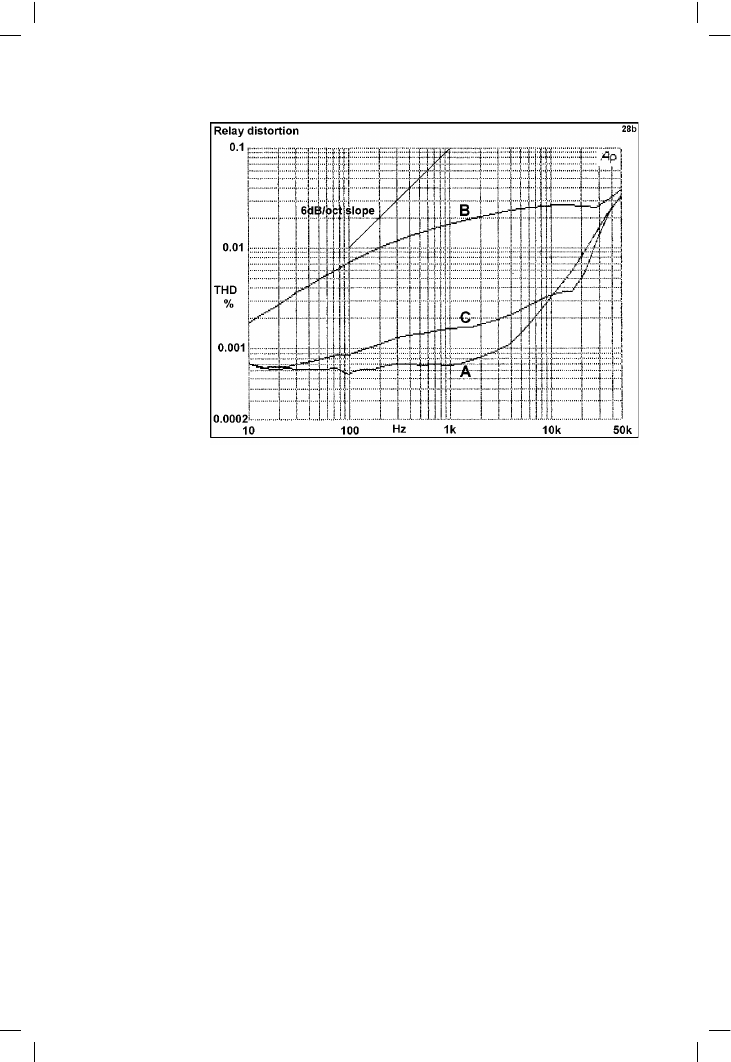
Audio Power Amplifier Design Handbook
Figure 13.13 shows the effect at 200 W rms/2 ! (i.e. with 10 A rms through
the load) before and after the relay. Trace A is the amplifier alone. This is a
Blameless amplifier and so THD is undetectable below 3 kHz, being
submerged in the noise floor which sets a measurement limit of 0.007%.
Trace B adds in the extra distortion from the relay. It seems to be frequency-
dependent, but rises more slowly than the usual slope of 6 dB/octave. Trace
C shows the effect of closing the relay in the NFB loop using the circuit and
component values of Figure 13.12; the THD drops to about a tenth, which
is what simple NFB theory would predict. Note that from 10 kHz to 35 kHz
the distortion is now lower than before the relay was added; this is due to
cancellation of amplifier and relay distortion.
Figure 13.14 was obtained by sawing a 3 mm by 15 mm piece from a relay
frame and wiring it in series with the amplifier output, by means of copper
wires soldered at each end. As before the level was 200 W rms/2 !, i.e. 10 A
rms. Trace A is the raw extra distortion; this is lower than shown in Figure
13.13 because the same current is passing through less of the frame
material. Trace B is the result of enclosing the frame fragment in the NFB
loop exactly as before. This removes all suspicion of interaction with coil
or contacts and proves it is the actual frame material itself that is non-
linear.
Wrapping feedback around the relay helps but, as usual, is not a complete
cure. Soldering on extra wires to the frame to bypass as much frame
material as possible is also useful, but it is awkward and there is the danger
of interfering with proper relay operation. No doubt any warranties would
390
Figure 13.13
A is amplifier
distortion alone, B
total distortion with
power relay in
circuit. C shows that
enclosing the relay in
the feedback loop is
not a complete cure
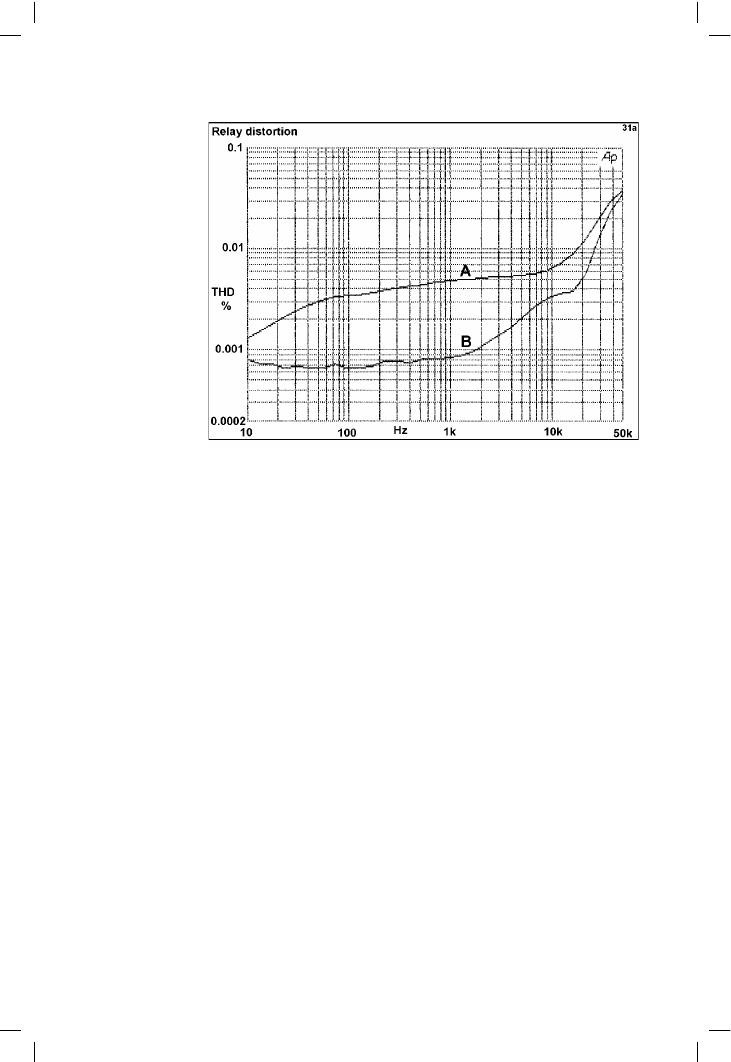
Amplifier and loudspeaker protection
be invalidated. Clearly it is best to avoid this sort of relay construction if you
possibly can, but if high-current switching is required, more than an audio-
intended relay can handle, the problem may have to be faced.
Output crowbar DC protection
Since relays are expensive and require control circuitry, and fuse protection
is very doubtful, there has for at least two decades been interest in simpler
and wholly solid-state solutions to the DC-protection problem. The circuit
of Figure 13.15 places a triac across the output, the output signal being
low-pass filtered by R and C. If sufficient DC voltage develops on C to fire
the diac, it triggers the triac, shorting the amplifier output to ground.
While this approach has the merit of simplicity, in my (wholly unhappy)
experience, it has proved unsatisfactory. The triac needs to be very big
indeed if it is to work more than once, because it must pass enough current
to blow the HT rail fuses. If these fuses were omitted the triac would have
to dump the entire contents of a power-supply reservoir capacitor to ground
through a low total resistance, and the demands on it become quite
unreasonable.
An output crowbar is also likely to destroy the output devices; the
assumption behind this kamikaze crowbar system is that the DC offset is due
to blown output devices, and a short across the output can do no more harm.
This is quite wrong, because any fault in the small-signal part of the amplifier
will also cause the output to saturate positive or negative, with the output
devices in perfect working order. The operation of the crowbar under these
391
Figure 13.14
Trace A here is total
distortion with a
sample of the power
relay frame material
wired in circuit. B is
the same, enclosed
in the feedback loop
as before
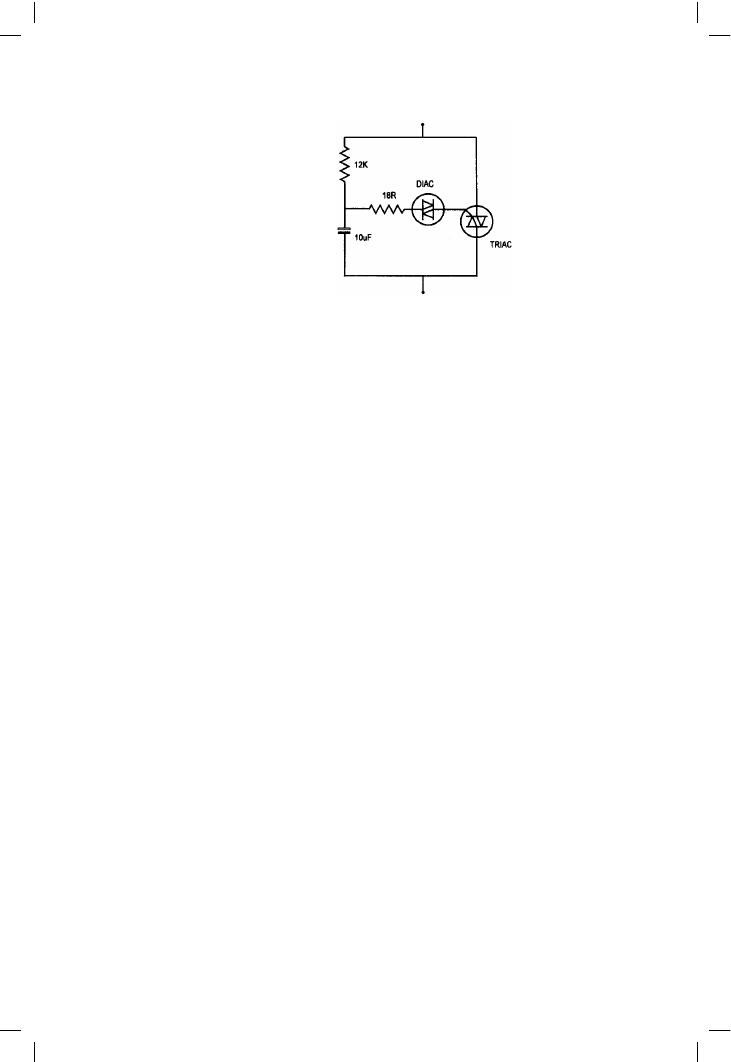
Audio Power Amplifier Design Handbook
circumstances may destroy the output devices, for the overload protection
may not be adequate to cope with such a very direct short-circuit.
Protection by power-supply shutdown
If your amplifier is powered by a switch-mode supply, it may well have a
logic input that gives the option of near-instant shutdown. This can be
connected to a DC-detect low-pass filter, and the occurrence of a DC error
then gives an apparently foolproof shutdown of everything.
There are (as usual) snags to this. Firstly, the high relative cost of switch-
mode supplies means that one will be shared between two or more
amplifier channels, and so both channels are lost if one fails. Secondly, and
more worryingly, this provides very dubious protection against a fault in the
supply itself. If such a fault causes one of the HT rails to collapse, then it
may well also disable the shutdown facility, and all protection is lost.
Conventional transformer power supplies can also be shut down quickly by
firing crowbar SCRs across the supply-rails; this overcomes one of the
objections to output crowbars, as collateral damage to other parts of the
circuit is unlikely, assuming of course you are correctly trying to blow the
DC rail fuses, and not the transformer secondary fuses. The latter option
would severely endanger the bridge rectifier, and the crowbar circuitry
would have to handle enormous amounts of energy as it emptied the
reservoir capacitors. Even blowing the DC fuses will require SCRs with a
massive peak-current capability.
Thermal protection
This section deals only with protecting the output semiconductors against
excessive junction temperature; the thermal safeguarding of the mains
transformer is dealt with in Chapter 8.
Output devices that are fully protected against excess current, voltage and
power are by no means fully safeguarded. Most electronic overload
protection systems allow the devices to dissipate much more power than in
392
Figure 13.15
Output crowbar DC
protection
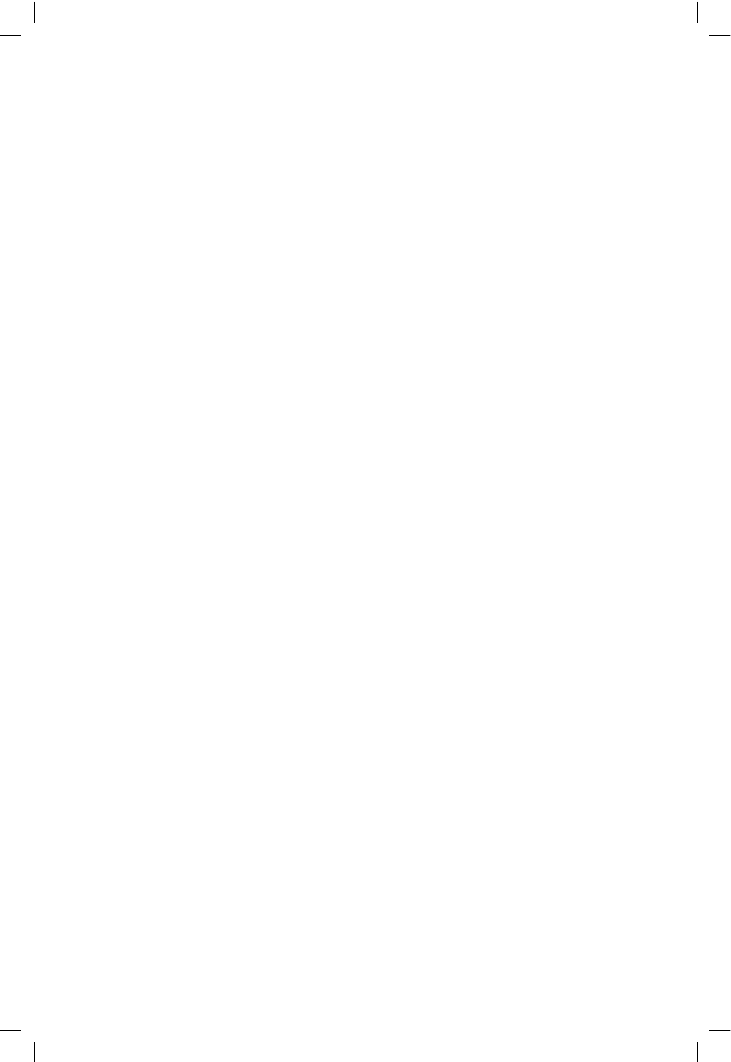
Amplifier and loudspeaker protection
normal operation; this can and should be well inside the rated capabilities
of the component itself, but this gives no assurance that the increased
dissipation will not cause the heatsink to eventually reach such tem-
peratures that the crucial junction temperatures are exceeded and the
device fails. If no temperature protection is provided this can occur after
only a few minutes drive into a short. Heatsink over-temperature may also
occur if ventilation slots, etc. are blocked, or heatsink fins covered up.
The solution is a system that senses the heatsink temperature and intervenes
when it reaches a preset maximum. This intervention may be in the form of:
1 Causing an existing muting/DC-protection relay to drop out, breaking
the output path to the load. If such a relay is fitted, then it makes sense to
use it.
2 Muting or attenuating the input signal so the amplifier is no longer
dissipating significant power.
3 Removing the power-supply to the amplifier sections. This normally
implies using a bimetallic thermal switch to break the mains supply to the
transformer primary, as anywhere downstream of here requires two lines
to be broken simultaneously, e.g. the positive and negative HT rails.
Each of these actions may be either self-resetting or latching, requiring the
user to initiate a reset. The possibility that a self-resetting system will cycle on
and off for long periods, subjecting the output semiconductors to severe
temperature changes, must be borne in mind. Such thermal cycling can
severely shorten the life of semiconductors.
The two essential parts of a thermal protection system are the temperature
sensing element and whatever arrangement performs the intervention.
While temperature can be approximately sensed in many ways, e.g. by
thermistors, silicon diodes, transistor junctions, etc. these all require some
sort of setup or calibration procedure, due to manufacturing tolerances. This
is impractical in production, for it requires the heatsink (which normally has
substantial thermal inertia) to be brought up to the critical temperature
before the circuit is adjusted. This not only takes considerable time, but also
requires the output devices to reach a temperature at which they are
somewhat endangered.
A much better method is the use of integrated temperature sensors that do not
require any calibration. A good example is the National Semiconductor
LM35DZ, a three-terminal TO92 device which outputs 10 mV for each
degree Centigrade above Zero. Without any calibration procedure, the
output voltage may be compared against a fixed reference, usually by an op-
amp used as a comparator, and the resulting output used to pull out the
muting relay. This approach gives the most trouble-free temperature
protection in my experience. IC temperature sensors are more expensive
than thermistors, etc. but this is counterbalanced by their accurate and
trouble-free operation.
393
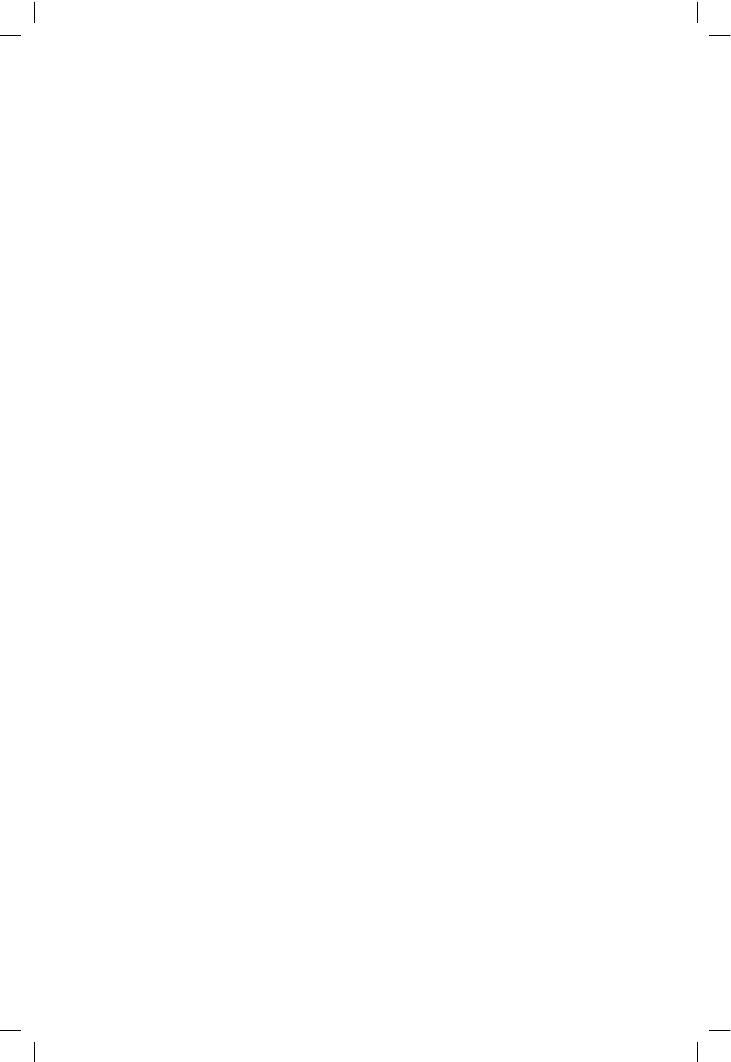
Audio Power Amplifier Design Handbook
Another pre-calibrated temperature sensor is the thermal switch, which
usually operates on the principle of a bistable bimetallic element. These
should not be confused with thermal fuses which are once-only components
that open the circuit by melting an internal fusible alloy; the trouble with
these is that they are relatively uncommon, and the chance of a blown
thermal fuse being replaced with the correct component in the field is not
high.
The physical positioning of the temperature sensor requires some thought. In
an ideal world we would judge the danger to the output devices by assessing
the actual junction temperature; since this is impractical the sensor must get
as close as it can. It is shown elsewhere that the top of a TO3 transistor can
gets hotter than the flange, and as for quiescent biasing sensors, the top is the
best place for the protection sensor. This does however present some
mechanical problems in mounting. This approach may not be equally
effective with plastic flat-pack devices such as TO3P, for the outer surface is
an insulator; however it still gets hotter than the immediately adjacent
heatsink.
Alternatively, the protection sensor can be mounted on the main heatsink,
which is mechanically much simpler but imposes a considerable delay
between the onset of device heating and the sensor reacting. For this reason a
heatsink-mounted sensor will normally need to be set to a lower trip
temperature, usually in the region of 80°C, than if it is device-mounted. The
more closely the sensor is mounted to the devices, the better they are
protected. If two amplifiers share the same heatsink, the sensor should be
placed between them; if it was placed at one end the remote amplifier would
suffer a long delay between the onset of excess heating and the sensor
acting.
One well-known make of PA amplifiers implements temperature protection
by mounting a thermal switch in the live mains line on top of one of the TO3
cans in the output stage. This gains the advantage of fast response to
dangerous temperatures, but there is the obvious objection that lethal
voltages are brought right into the centre of the amplifier circuitry, where
they are not normally expected, and this represents a real hazard to service
personnel.
Powering auxiliary circuitry
Whenever it is necessary to power auxiliary circuitry, such as the relay
control system described above, there is an obvious incentive to use the
main HT rails. A separate PSU requires a bridge rectifier, reservoir capacitor,
fusing and an extra transformer winding, all of which will cost a significant
amount of money.
The main disadvantage is that the HT rails are at an inconveniently high
voltage for powering control circuitry. For low-current sections of this
394