ВУЗ: Казахская Национальная Академия Искусств им. Т. Жургенова
Категория: Учебное пособие
Дисциплина: Не указана
Добавлен: 03.02.2019
Просмотров: 17380
Скачиваний: 18
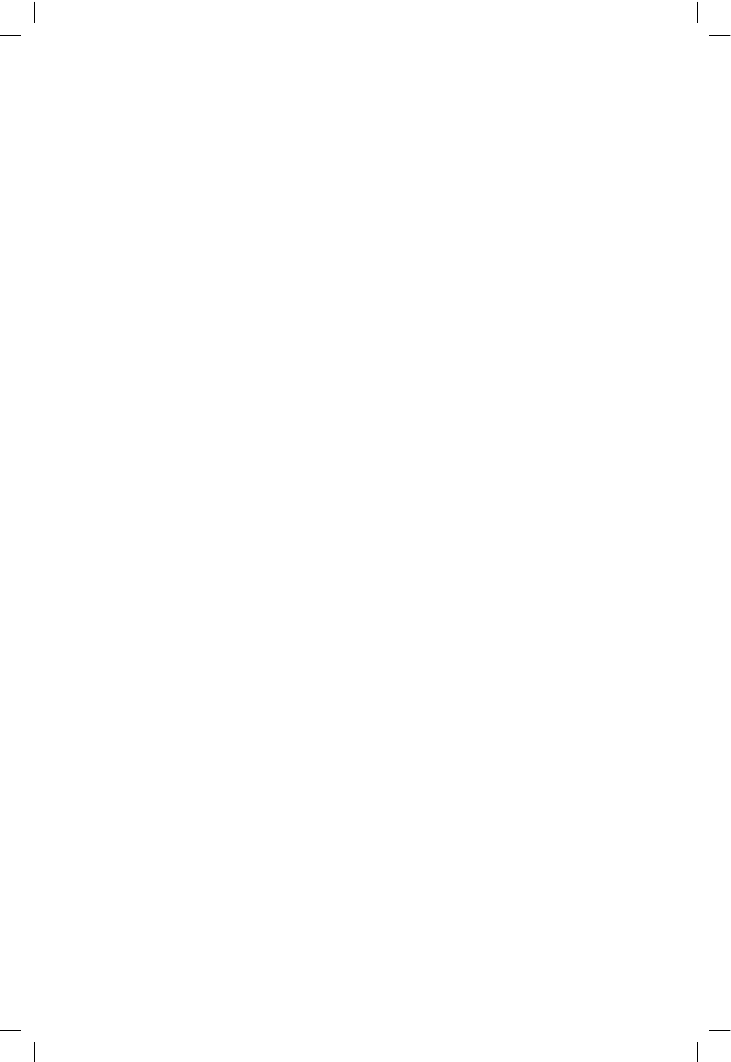
The small signal stages
across two base-emitter junctions in series, one in each arm of the circuit.
In theory the errors due to non-linear re of the transistors is divided by beta,
but in practice the reduction in distortion is modest.
The cascomp (Figure 4.10c) does not have problems with negative
impedances, but it is significantly more complex to design. Q2, Q3 are the
main input pair as before, delivering current through cascode transistors
Q4, Q5 (this does not in itself affect linearity), which, since they carry
almost the same current as Q2, Q3 duplicate the input Vbe errors at their
emitters. This is sensed by error diff-amp Q6, Q7, whose output currents
are summed with the main output in the correct phase for error-correction.
By careful optimisation of the (many) circuit variables, distortion at –30 dBu
input can be reduced to about 0.016% with the circuit values shown. Sadly,
this effort provides very little further improvement in whole-amplifier HF
distortion over the simpler CFP input, as other distortion mechanisms are
coming into play, one of which is the finite ability of the VAS to source
current into the other end of Cdom.
Input stage cascode configurations
Power amplifiers with pretensions to sophistication sometimes add
cascoding to the standard input differential amplifier. This does nothing
whatever to improve input-stage linearity, as there is no appreciable voltage
swing on the input collectors; its main advantage is reduction of the high
Vce that the input devices work at. This allows cooler running, and
therefore possibly improved thermal balance; a Vce of 5 V usually works
well. Isolating the input collector capacitance from the VAS input
sometimes allows Cdom to be slightly reduced for the same stability
margins, but the improvement is marginal.
85
Input noise and how to reduce it
The noise performance of a power amplifier is defined by its input stage,
and so the issue is examined here. Power-amp noise is not an irrelevance;
a powerful amplifier will have a high voltage gain, and this can easily result
in a faint but irritating hiss from efficient loudspeakers even when all
volume controls are fully retarded
[3]
. In the design considered here the EIN
has been measured at –120 dBu, which is only 7 or 8 dB worse than a first-
class microphone preamplifier; the inferiority is largely due to the source
resistances seen by the input devices being higher than the usual 150 !
microphone impedance. By way of demonstration, halving the impedance
of the usual feedback network (22k and 1k) reduces the EIN further by
about 2 dB.
Amplifier noise is defined by a combination of the active devices at the
input and the surrounding resistances. The operating conditions of the
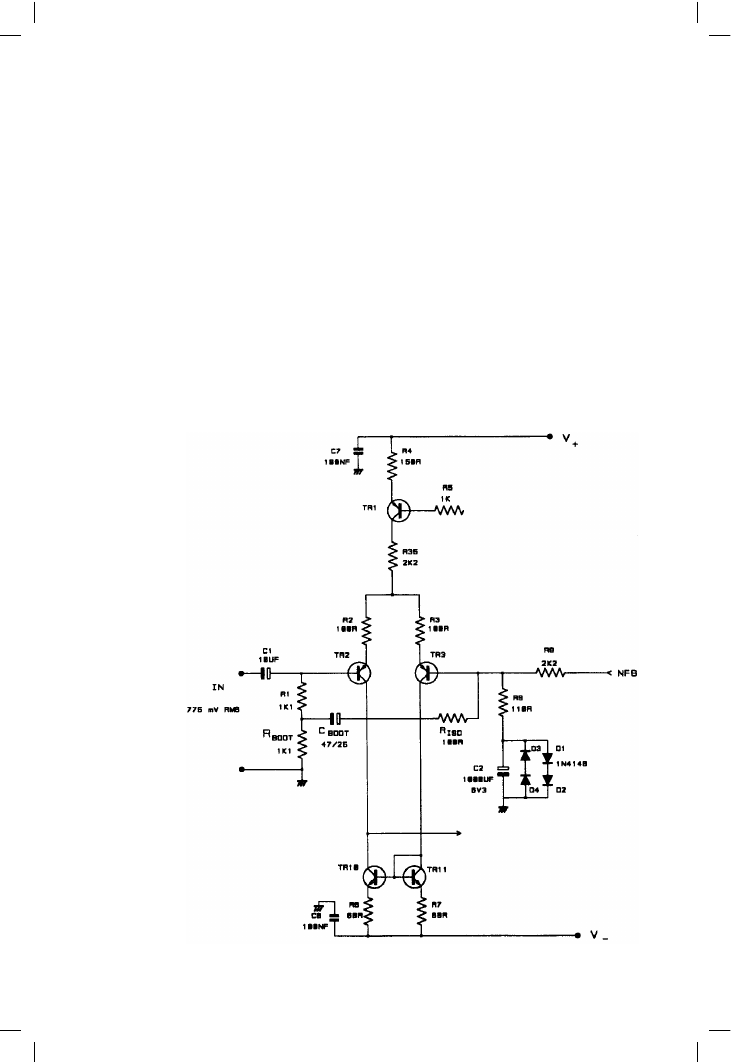
Audio Power Amplifier Design Handbook
input transistors themselves are set by the demands of linearity and slew-
rate, so there is little freedom of design here; however the collector currents
are already high enough to give near-optimal noise figures with the low
source impedances (a few hundred ohms) that we have here, so this is not
too great a problem. Noise figure is a weak function of Ic, so minor
tweakings of the tail-current make no detectable difference. We certainly
have the choice of input device type; there are many more possibles if we
have relatively low rail voltages. Noise performance is, however, closely
bound up with source impedance, and we need to define this before device
selection.
Looking therefore to the passives, there are several resistances generating
Johnson Noise in the input, and the only way to reduce this noise is to
reduce them in value. The obvious candidates are R2, R3 see Figure 4.12
(input stage degeneration resistors) and R9, which determines the output
86
Figure 4.12
Stable input
bootstrapping from the
feedback point. Riso is
essential for HF
stability; with 100 !,
as shown, the input
impedance is 13 k!
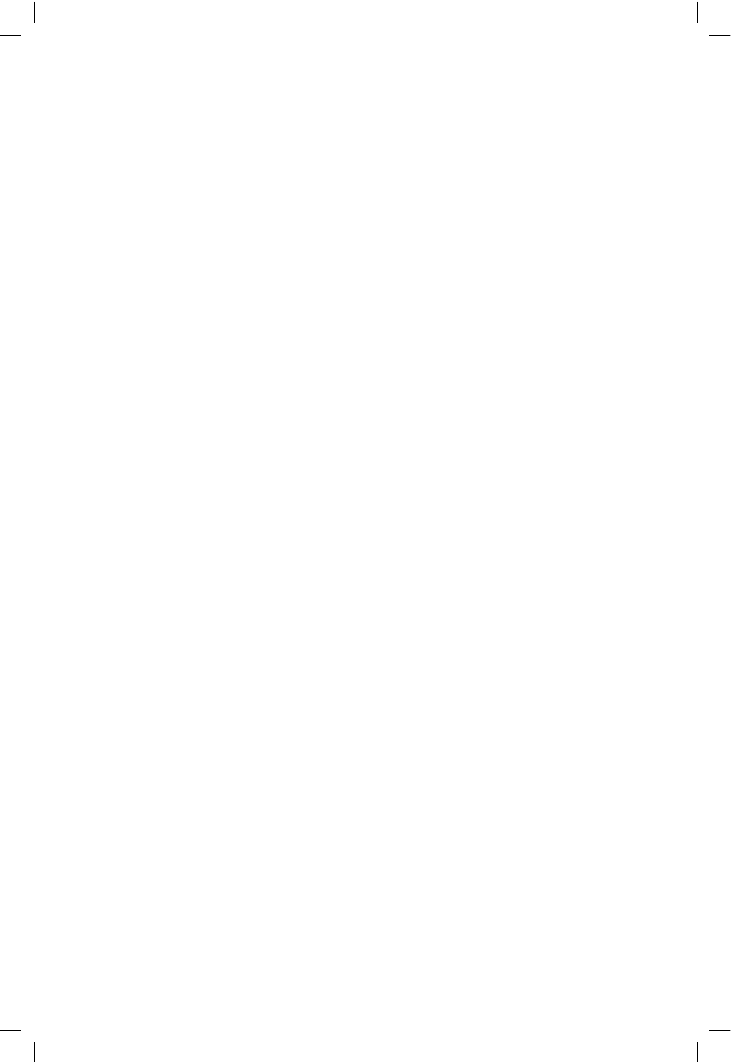
The small signal stages
impedance of the negative-feedback network. There is also another unseen
component; the source resistance of the preamplifier or whatever upstream.
Even if this equipment were miraculously noise-free, its output resistance
would still generate Johnson noise. If the preamplifier had, say, a 20k
volume pot at its output (not a good idea, as this gives a poor gain structure
and cable dependent HF losses, but that is another story
[5]
) then the source
resistance could be a maximum of 5k, which would almost certainly
generate enough Johnson Noise to dominate the power-amplifier’s noise
behaviour. However, there is nothing that power-amp designers can do
about this, so we must content ourselves with minimising the noise-
generating resistances we do have control over.
Noise from the input degeneration resistors R2, R3 is the price we pay for
linearising the input stage by running it at a high current, and then bringing
its transconductance down to a useable value by adding linearising local
negative feedback. These resistors cannot be reduced if the HF NFB factor
is then to remain constant, for Cdom will have to be proportionally
increased, reducing slew-rate. With the original 22k–1k NFB network,
these resistors degrade the noise performance by 1.7 dB. (This figure, like
all other noise measurements given here, assumes a 50 ! external source
resistance.)
If we cannot alter the input degeneration resistors, then the only course left
is the reduction of the NFB network impedance, and this sets off a whole
train of consequences. If R8 is reduced to 2k2, then R9 becomes 110 !, and
this reduces noise output from –93.5 dBu to –95.4 dBu. (Note that if R2, R3
were not present, the respective figures would be –95.2 and –98.2 dBu.)
However, R1 must also be reduced to 2k2 to maintain DC balance, and this
is too low an input impedance for direct connection to the outside world.
If we accept that the basic amplifier will have a low input impedance, there
are two ways to deal with it. The simplest is to decide that a balanced line
input is essential; this puts an op-amp stage before the amplifier proper,
buffers the low input impedance, and can provide a fixed source
impedance to allow the HF and LF bandwidths to be properly defined by
an RC network using non-electrolytic capacitors. The usual practice of
slapping an RC network on an unbuffered amplifier input must be roundly
condemned as the source impedance is unknown, and so therefore is the
roll-off point. A major stumbling block for subjectivist reviewing, one
would have thought.
Another approach is to have a low resistance DC path at the input but a
high AC impedance; in other words to use the fine old practice of input
bootstrapping. Now this requires a low-impedance unity-gain-with-
respect-to-input point to drive the bootstrap capacitor, and the only one
available is at the amplifier inverting input, i.e. the base of TR3. While this
node has historically been used for the purpose of input bootstrapping
[6]
, it
has only been done with simple circuitry employing very low feedback
87
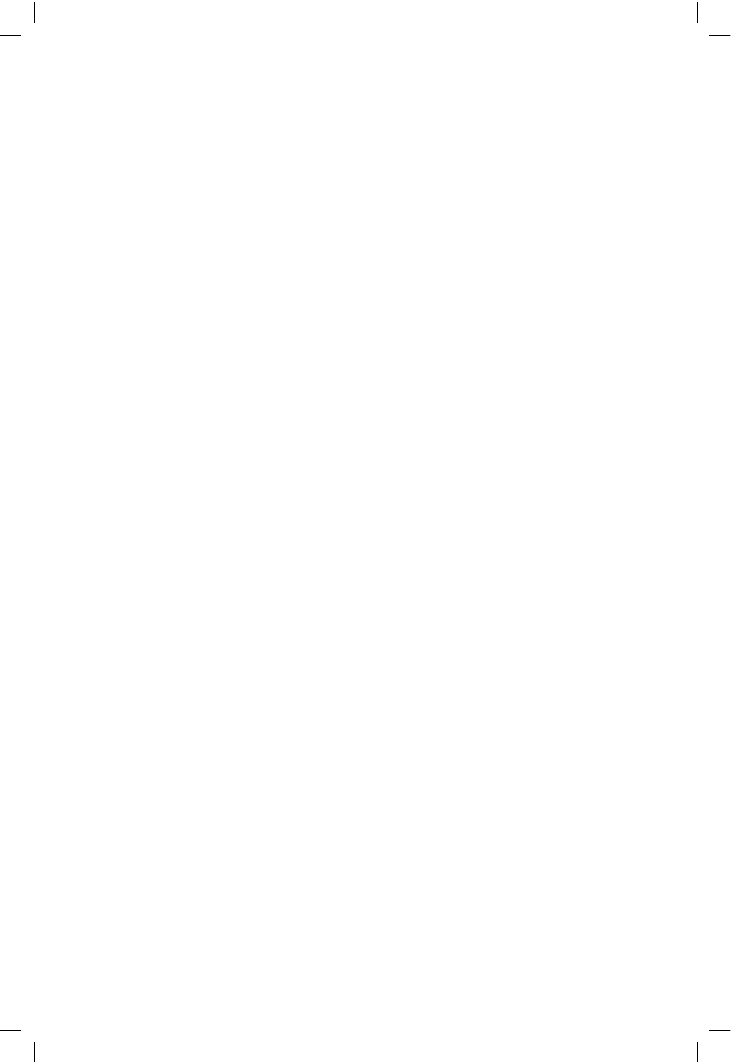
Audio Power Amplifier Design Handbook
factors. There is very real reason to fear that any monkey business with the
feedback point (TR3 base) will add shunt capacitance, creating a feedback
pole that will degrade HF stability. There is also the awkward question of
what will happen if the input is left open-circuit . . .
The input can be safely bootstrapped; Figure 4.12 shows how. The total DC
resistance of R1 and Rboot equals R8, and their central point is driven by
Cboot. Connecting Cboot directly to the feedback point did not produce
gross instability, but it did seem to increase susceptibility to odd bits of
parasitic oscillation. Riso was then added to isolate the feedback point from
stray capacitance, and this seemed to effect a complete cure. The input
could be left open-circuit without any apparent ill-effects, though this is not
good practice if loudspeakers are connected. A value for Riso of 220 !
increases the input impedance to 7.5k, and 100 ! raises it to 13.3k, safely
above the 10k standard value for a bridging impedance. Despite successful
tests, I must admit to a few lingering doubts about the HF stability of this
approach, and it might be as well to consider it as experimental until more
experience is gained.
One more consequence of a low-impedance NFB network is the need for
feedback capacitor C2 to be proportionally increased to maintain LF
response, and prevent capacitor distortion from causing a rise in THD at
low frequencies; it is the latter requirement that determines the value. (This
is a separate distortion mechanism from the seven originally identified, and
is given the title Distortion 8.) This demands a value of 1000 µF,
necessitating a low rated voltage such as 6V3 if the component is to be of
reasonable size. This means that C2 needs protective shunt diodes in both
directions, because if the amplifier fails it may saturate in either direction.
Examination of the distortion residual shows that the onset of conduction of
back-to-back diodes will cause a minor increase in THD at 10 Hz, from less
than 0.001% to 0.002%, even at the low power of 20 W/8 !. It is not my
practice to tolerate such gross non-linearity, and therefore four diodes are
used in the final circuit, and this eliminates the distortion effect. It could be
argued that a possible reverse-bias of 1.2 V does not protect C2 very well,
but at least there will be no explosion.
We can now consider alternative input devices to the MPSA56, which was
never intended as a low-noise device. Several high-beta low-noise types
such as 2SA970 give an improvement of about 1.8 dB with the low-
impedance NFB network. Specialised low-Rb devices like 2SB737 give
little further advantage (possibly 0.1 dB) and it is probably better to go for
one of the high-beta types; the reason why will soon emerge.
It could be argued that the above complications are a high price to pay for
a noise reduction of some 2 dB; however, with the problems comes a
definite advantage, for the above NFB network modification also sig-
nificantly improves the output DC offset performance.
88
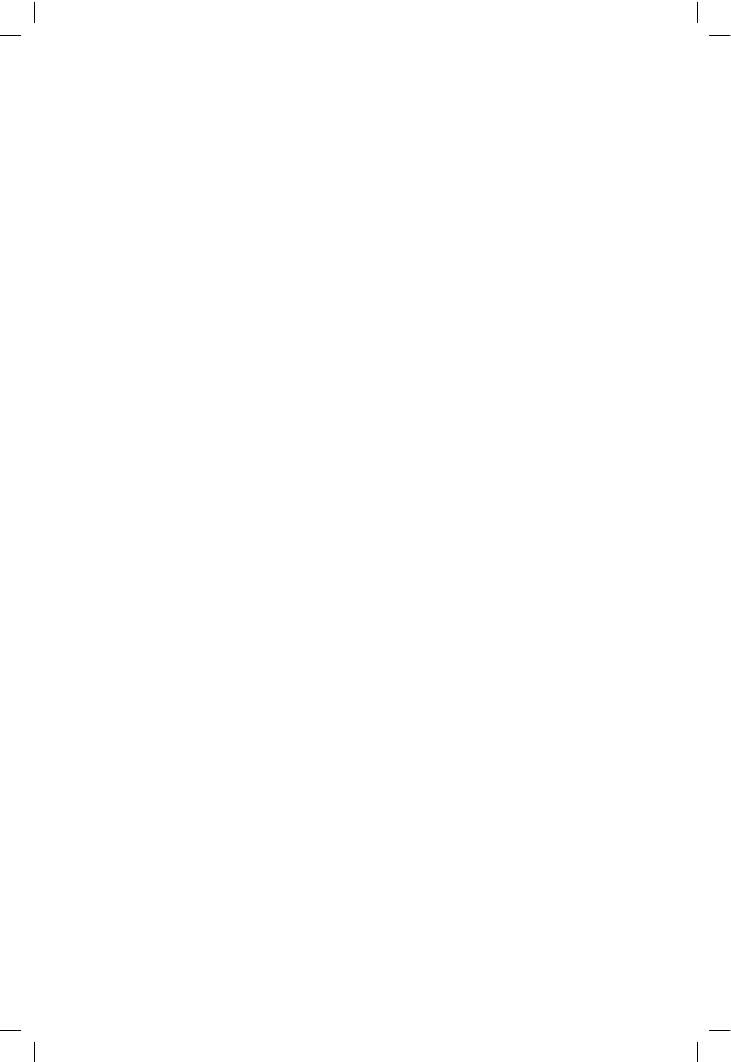
The small signal stages
Offset and match: the DC precision issue
The same components that dominate amplifier noise performance also
determine the output DC offset; if R9 is reduced to minimise the source
resistance seen by TR3, then the value of R8 is scaled to preserve the same
closed-loop gain, and this reduces the voltage drops caused by input
transistor base currents.
Most of my amplifier designs have assumed that a +/–50 mV output DC
offset is acceptable. This allows DC trimpots, offset servos, etc. to be
gratefully dispensed with. However, it is not in my nature to leave well
enough alone, and it could be argued that +/–50 mV is on the high side for
a top-flight amplifier. I have therefore reduced this range as much as
possible without resorting to a servo; the required changes have already
been made when the NFB network was reduced in impedance to minimise
Johnson noise. (See page 85.)
With the usual range of component values, the DC offset is determined not
so much by input transistor Vbe mismatch, which tends to be only 5 mV or
so, but more by a second mechanism – imbalance in beta. This causes
imbalance of the base currents (Ib) drawn thorough input bias resistor R1
and feedback resistor R8, and the cancellation of the voltage-drops across
these components is therefore compromised.
A third source of DC offset is non-ideal matching of input degeneration
resistors R2, R3. Here they are 100 !, with 300 mV dropped across each, so
two 1% components at opposite ends of their tolerance bands could give
a maximum offset of 6 mV. In practice this is most unlikely, and the error
from this source will probably not exceed 2 mV.
There are several ways to reduce DC offset. Firstly, low-power amplifiers
with a single output pair must be run from modest HT rails and so the
requirement for high-Vce input transistors can be relaxed. This allows
higher beta devices to be used, directly reducing Ib. The 2SA970 devices
used in this design have a beta range of 350–700, compared with 100 or
less for MPSA06/56. Note the pinout is not the same.
On page 85, we reduced the impedance of the feedback network by a
factor of 4.5, and the offset component due to Ib imbalance is reduced by
the same ratio. We might therefore hope to keep the DC output offset for
the improved amplifier to within +/–15 mV without trimming or servos.
Using high-beta input devices, the Ib errors did not exceed +/–15 mV for
ten sample pairs (not all from the same batch) and only three pairs
exceeded +/–10 mV. Ib errors are now reduced to the same order of
magnitude as Vbe mismatches, and so no great improvement can be
expected from further reduction of circuit resistances. Drift over time was
measured at less than 1 mV, and this seems to be entirely a function of
temperature equality in the input pair.
89