ВУЗ: Казахская Национальная Академия Искусств им. Т. Жургенова
Категория: Учебное пособие
Дисциплина: Не указана
Добавлен: 03.02.2019
Просмотров: 17400
Скачиваний: 18
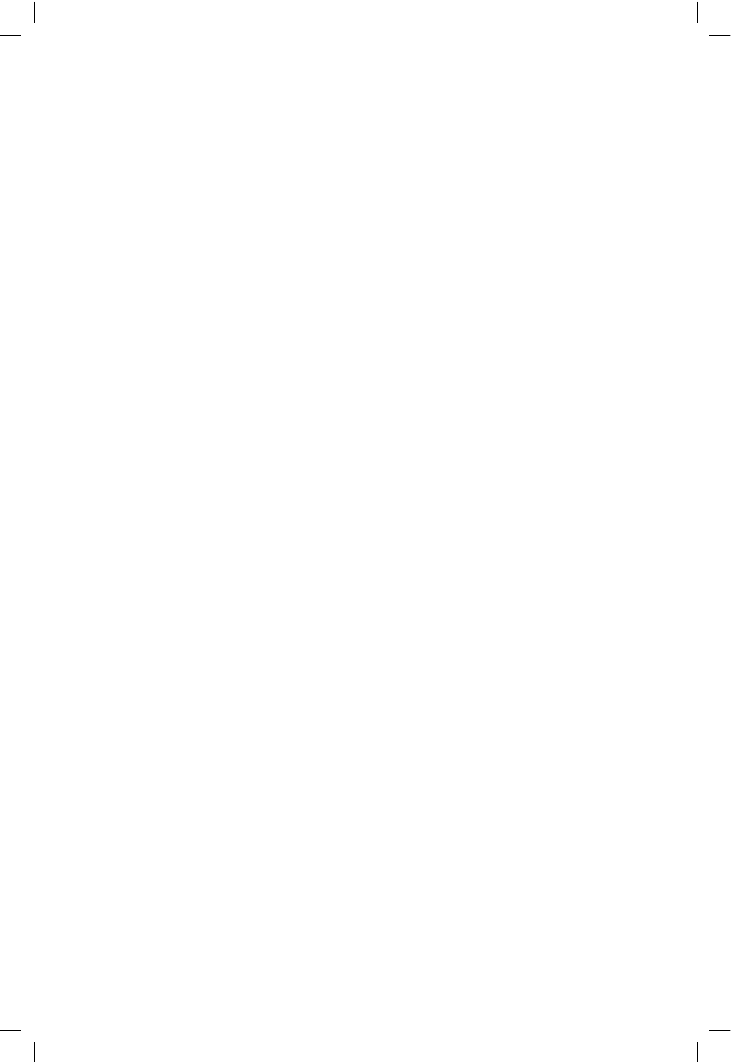
Audio Power Amplifier Design Handbook
output devices to lay down their lives to save the rail fuses. The tail-source
TR17 is particularly vulnerable because any fault that extinguishes the tail-
current removes the drive to TR14, the controller is disabled, and the
current in the output stage will be very large. In Figure 9.18 the Vbe-
multiplier TR13 acts as a safety-circuit which limits Vbias to about 600 mV
rather than the normal 300 mV, even if the current-controller is completely
non-functional and TR14 fully off. This gives a quiescent of 3.0 A, and I can
testify this is a survivable experience for the output devices in the short-
term; however they may eventually fail from overheating if the condition is
allowed to persist.
There are some important points about the current-controller. The entire
tail-current for the error-amplifier, determined by TR17, is syphoned off
from VAS current source TR5, and must be taken into account when
ensuring that the upper output half gets enough drive current.
There must be enough tail-current available to turn on TR14, remembering
that most of TR16 collector-current flows through R15, to keep the pair
roughly balanced. If you feel moved to alter the VAS current, remember
also that the base current for driver TR6 is higher in Class-A than Class-B,
so the positive slew-rate is slightly reduced in going from Class-A to B.
The original Class-A amplifier used a National LM385/1.2, its output
voltage fixed at 1.223 V nominal; this was reduced to approx. 0.6 V by a
1k–1k divider. The circuit also worked well with Vref provided by a silicon
diode, 0.6 V being an appropriate Vbias drop across two 0.22 ! output
emitter resistors. This is simple, and retains the immunity of Iq to heatsink
and output device temperatures, but it does sacrifice the total immunity to
ambient temperature that a band-gap reference gives.
The LM385/1.2 is the lowest voltage band-gap reference commonly
available; however, the voltages shown in Figure 9.18 reveal a difficulty
with the new lower Vbias value and the CFP stage; points A and Y are now
only 960 mV apart, which does not give the reference room to work in if it
is powered from node A, as in the original circuit. The solution is to power
the reference from the V+ rail, via R42 and R43. The mid-point of these two
resistors is bootstrapped from the amplifier output rail by C5, keeping the
voltage across R43 effectively constant. Alternatively, a current-source
could be used, but this might reduce positive headroom. Since there is no
longer a strict upper limit on the reference voltage, a more easily obtainable
2.56 V device could be used providing R30 is suitably increased to 5k to
maintain Vref at 300 mV across R31.
In practical use, Iq stability is very good, staying within 1% for long periods.
The most obvious limitation on stability is differential heating of TR15, 16
due to heat radiation from the main heatsink. TR14 should also be sited
with this in mind, as heating it will increase its beta and slightly imbalance
TR15, 16.
280
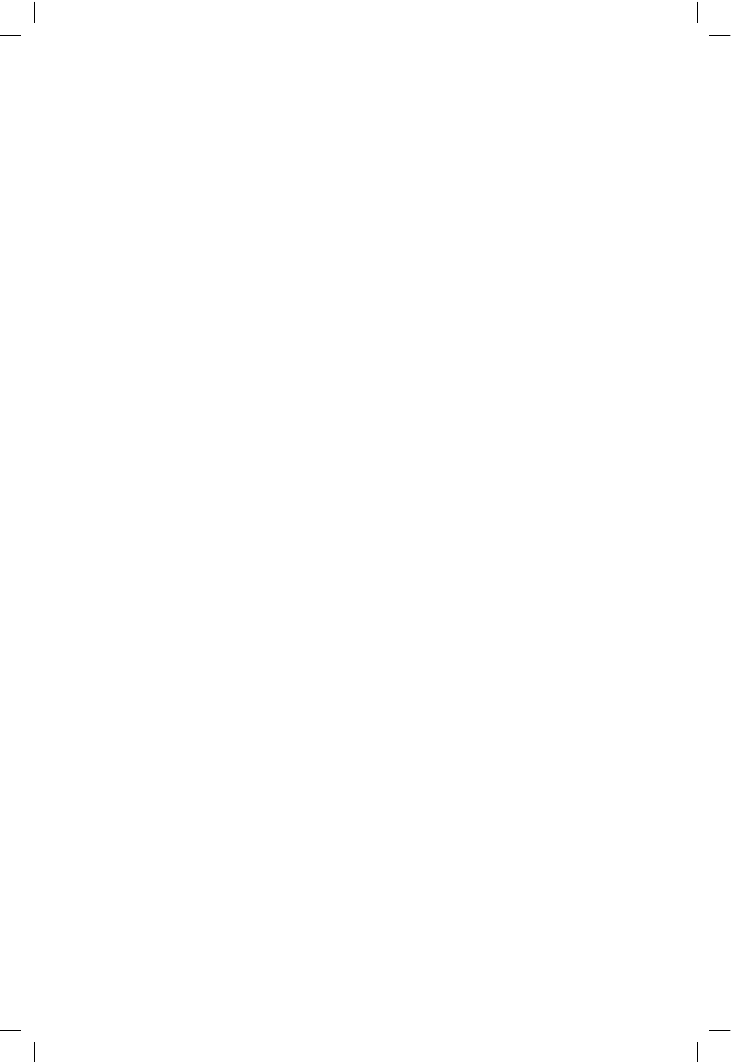
Class-A power amplifiers
Class-B mode
In Class-B mode, the current-controller is disabled, by turning off tail-
source TR17 so TR14 is firmly off, and critical biasing for minimal crossover
distortion is provided as usual by Vbe-multiplier TR13. With 0.1 ! emitter
resistors Vbias (between X and Y) is approx. 10 mV. I would emphasise that
in Class-B this design, if constructed correctly, will be as Blameless as a
purpose-built Class-B amplifier. No compromises have been made in
adding the mode-switching.
As in the previous Class-B design, the addition of R14 to the Vbe-multiplier
compensates against drift of the VAS current-source TR5. To make an old
but much-neglected point, the preset should always be in the bottom arm
of the Vbe divider R10, 11, because when presets fail it is usually by the
wiper going open; in the bottom arm this gives minimum Vbias, but in the
upper it would give maximum.
In Class-B, temperature compensation for changes in driver dissipation
remains vital. Thermal runaway with the CFP is most unlikely, but accurate
quiescent setting is the only way to minimise cross-over distortion. TR13 is
therefore mounted on the same small heatsink as driver TR6. This is often
called thermal feedback, but it is no such thing as TR13 in no way controls
the temperature of TR6; thermal feedforward would be a more accurate
term.
The mode-switching system
The dual nature of the biasing system means Class-A/Class-B switching can
be implemented fairly simply. A Class-A amplifier is an uneasy companion
in hot weather, and so I have been unable to resist the temptation to sub-
title the mode switch Summer/Winter, by analogy with a car air intake.
The switchover is DC-controlled, as it is not desirable to have more signal
than necessary running around inside the box, possibly compromising
interchannel crosstalk. In Class-A/AB mode, SW1 is closed, so TR17 is
biased normally by D5, 6, and TR20 is held on via R33, shorting out preset
PR1 and setting TR13 to safety mode, maintaining a maximum Vbias limit
of 600 mV. For Class-B, SW1 is opened, turning off TR17 and therefore
TR15, 16 and 14. TR20 also ceases to conduct, protected against reverse-
bias by D9, and reduces the voltage set by TR13 to a suitable level for
Class-B. The two control pins of a stereo amplifier can be connected
together, and the switching performed with a single-pole switch, without
interaction or increased crosstalk.
The mode-switching affects the current flowing in the output devices, but
not the output voltage, which is controlled by the global feedback loop,
and so it is completely silent in operation. The mode may be freely
switched while the amplifier is handling audio, which allows some
interesting A/B listening tests.
281
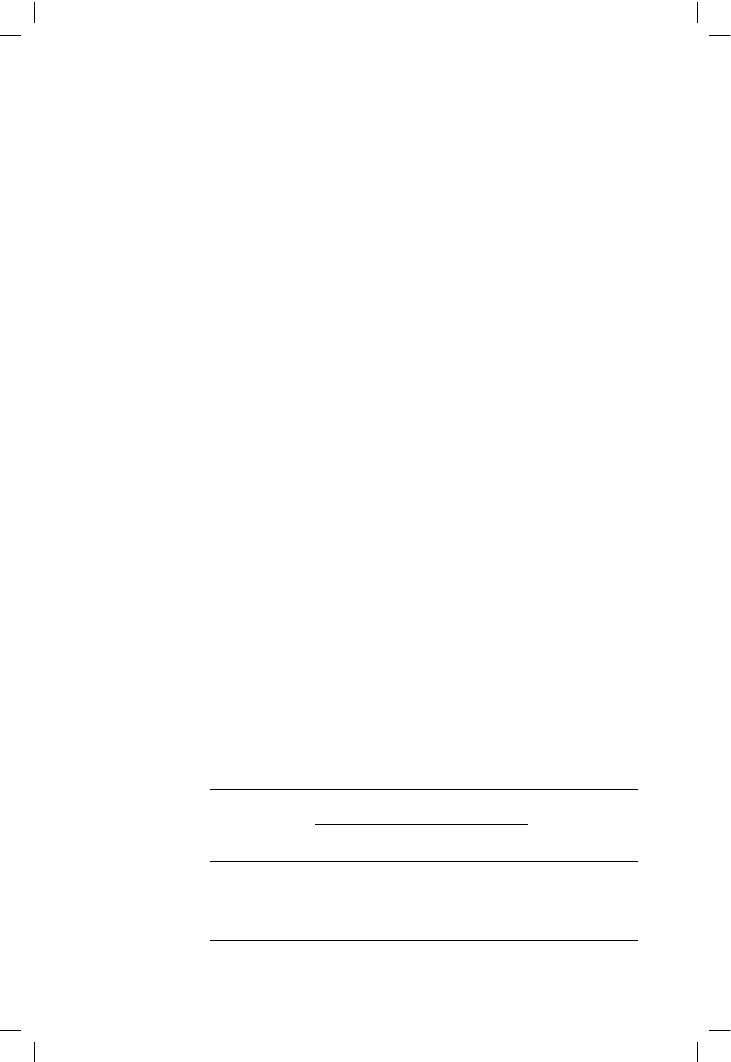
Audio Power Amplifier Design Handbook
It may be questioned why it is necessary to explicitly disable the current-
controller in Class-B; TR13 is establishing a lower voltage than the current-
controller which latter subsystem will therefore turn TR14 off as it strives
futilely to increase Vbias. This is true for 8 ! loads, but 4 ! impedances
increase the currents flowing in R16, 17 so they are transiently greater than
the Class-A Iq, and the controller will therefore intermittently take control
in an attempt to reduce the average current to 1.5 A. Disabling the
controller by turning off TR17 via R44 prevents this.
If the Class-A controller is enabled, but the preset PR1 is left in circuit (e.g.
by shorting TR20 base-emitter) we have a test mode which allows suitably
cautious testing; Iq is zero with the preset fully down, as TR13 over-rides
the current-controller, but increases steadily as PR1 is advanced, until it
suddenly locks at the desired quiescent current. If the current-controller is
faulty then Iq continues to increase to the defined maximum of 3.0 A.
Thermal design
Class-A amplifiers are hot almost by definition, and careful thermal design
is needed if they are to be reliable, and not take the varnish off the
Sheraton. The designer has one good card to play; since the internal
dissipation of the amplifier is maximal with no signal, simply turning on the
prototype and leaving it to idle for several hours will give an excellent idea
of worst-case component temperatures. In Class-B the power dissipation is
very program-dependent, and estimates of actual device temperatures in
realistic use are notoriously hard to make.
Table 9.5 shows the output power available in the various modes, with
typical transformer regulation, etc.; the output mode diagram in Figure 9.11
shows exactly how the amplifier changes mode from A to AB with
decreasing load resistance. Remember that in this context high distortion
means 0.002% at 1 kHz. This diagram was produced in the analysis section
of PSpice simply by typing in equations, and without actually simulating
anything at all.
The most important thermal decision is the size of the heatsink; it is going
to be expensive, so there is a powerful incentive to make it no bigger than
282
Table 9.5
Power capability
Load resistance
8 !
6 !
4 !
Distortion
Class-A
20 W
27 W
15 W
Low
Class-AB
n/a
n/a
39 W
High
Class-B
21 W
28 W
39 W
Medium
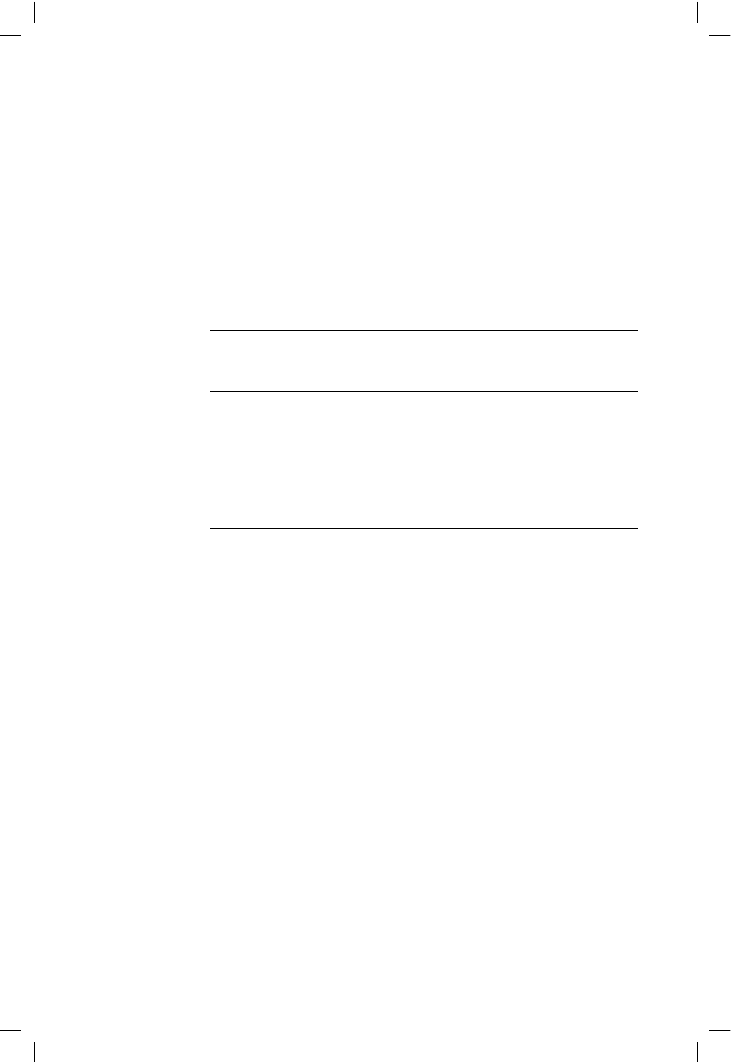
Class-A power amplifiers
necessary. I have ruled out fan cooling as it tends to make concern for ultra-
low electrical noise look rather foolish; let us rather spend the cost of the
fan on extra cooling fins and convect in ghostly silence. The exact thermal
design calculations are simple but tedious, with many parameters to enter;
the perfect job for a spreadsheet. The final answer is the margin between
the predicted junction temperatures and the rated maximum. Once power
output and impedance range are decided, the heatsink thermal resistance
to ambient is the main variable to manipulate; and this is a compromise
between coolness and cost, for high junction temperatures always reduce
semiconductor reliability. Looking at it very roughly:
This shows that the transistor junctions will be 80 degrees above ambient,
i.e. at around 100°C; the rated junction maximum is 200°C, but it really
isn’t wise to get anywhere close to this very real limit. Note the Case-Sink
thermal washers were high-efficiency material, and standard versions have
a slightly higher thermal resistance.
The heatsinks used in the prototype had a thermal resistance of 0.65°C/W
per channel. This is a substantial chunk of metal, and since aluminium is
basically congealed electricity, it’s bound to be expensive.
A complete Trimodal amplifier circuit
The complete Class-A amplifier is shown in Figure 9.19, complete with
optional input bootstrapping. It may look a little complex, but we have only
added four low-cost transistors to realise a high-accuracy Class-A quiescent
controller, and one more for mode-switching. Since the biasing system has
been described above, only the remaining amplifier subsystems are dealt
with here.
The input stage follows my design methodology by using a high tail current
to maximise transconductance, and then linearising by adding input
degeneration resistors R2, 3 to reduce the final transconductance to a
283
Thermal resistance
°C/W
Heat flow
Watts
Temp rise
°C
Temp
°C
100 junction
Juncn to TO3 Case
0.7
36 W
25
75 TO3 case
Case to Sink
0.23
36 W
8
67 Heatsink
Sink to air
0.65
72 W
47
20 Ambient
Total
80
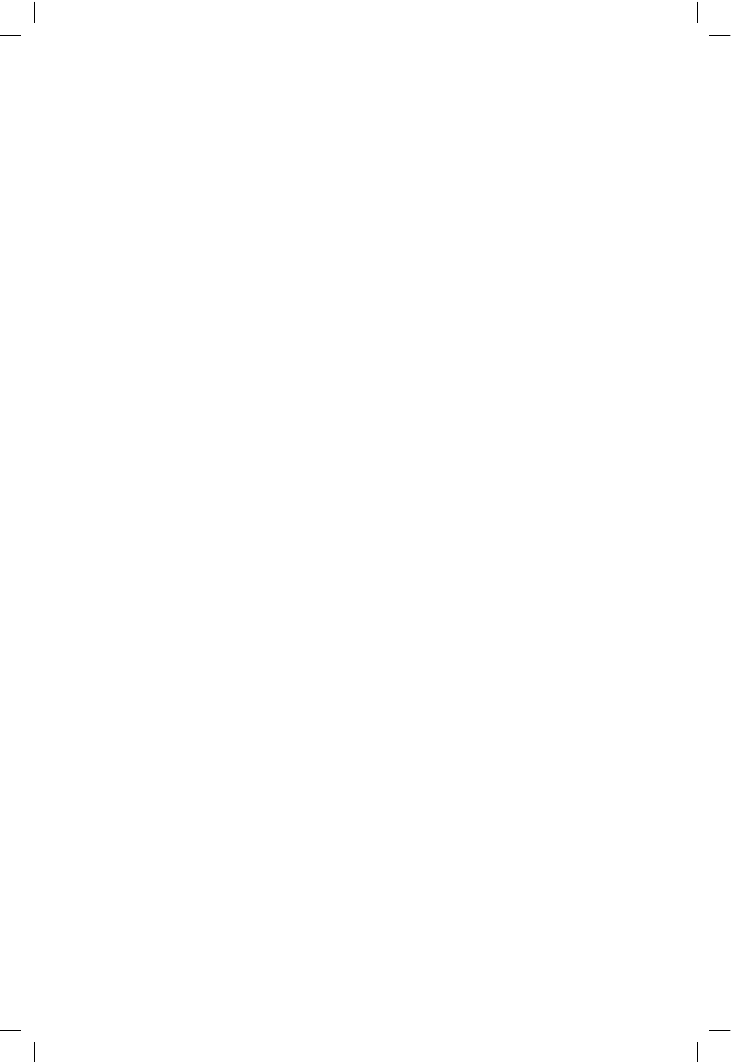
Audio Power Amplifier Design Handbook
suitable level. Current-mirror TR10, 11 forces the collector currents of the
two input devices TR2, 3 to be equal, balancing the input stage to prevent
the generation of second-harmonic distortion. The mirror is degenerated by
R6, 7 to eliminate the effects of Vbe mismatches in TR10, 11. With some
misgivings I added the input network R9, C15, which is definitely not
intended to define the system bandwidth, unless fed from a buffer stage;
with practical values the HF roll-off could vary widely with the source
impedance driving the amplifier. It is intended rather to give the possibility
of dealing with RF interference without having to cut tracks. R9 could be
increased for bandwidth definition if the source impedance is known, fixed,
and taken into account when choosing R9; bear in mind that any value
over 47 ! will measurably degrade the noise performance. The values
given roll off above 150 MHz to keep out UHF.
The input-stage tail current is increased from 4 to 6 mA, and the VAS
standing current from 6 to 10 mA over the original Chapter 6 circuit. This
increases maximum positive and negative slew-rates from +21, –48 V/µsec
to +37, –52 V/µsec; as described in Chapter 7, this amplifier architecture is
bound to slew asymmetrically. One reason is feedthrough in the VAS
current source; in the original circuit an unexpected slew-rate limit was set
by fast edges coupling through the current-source c-b capacitance to
reduce the bias voltage during positive slewing. This effect is minimised
here by using the negative-feedback type of current source bias generator,
with VAS collector current chosen as the controlled variable. TR21 senses
the voltage across R13, and if it attempts to exceed Vbe, turns on further to
pull up the bases of TR1 and TR5. C11 filters the DC supply to this circuit
and prevents ripple injection from the V+ rail. R5, C14 provide decoupling
to prevent TR5 from disturbing the tail-current while controlling the VAS
current.
The input tail-current increase also slightly improves input-stage linearity,
as it raises the basic transistor gm and allows R2, 3 to apply more local
NFB.
The VAS is linearised by beta-enhancing stage TR12, which increases
the amount of local NFB through Miller dominant-pole capacitor C3
(i.e. Cdom). R36 has been increased to 2k2 to minimise power dissipation,
as there seems no significant effect on linearity or slewing. Do not omit it
altogether, or linearity will be affected and slewing much compromised.
As described in Chapter 8, the simplest way to prevent ripple from entering
the VAS via the V– rail is old-fashioned RC decoupling, with a small R and
a big C. We have some 200 mV in hand (see page 274) in the negative
direction, compared with the positive, and expending this as the voltage-
drop through the RC decoupling will give symmetrical clipping. R37 and
C12 perform this function; the low rail voltages in this design allow the
1000 µF C12 to be a fairly compact component.
284