ВУЗ: Казахская Национальная Академия Искусств им. Т. Жургенова
Категория: Учебное пособие
Дисциплина: Не указана
Добавлен: 03.02.2019
Просмотров: 17399
Скачиваний: 18
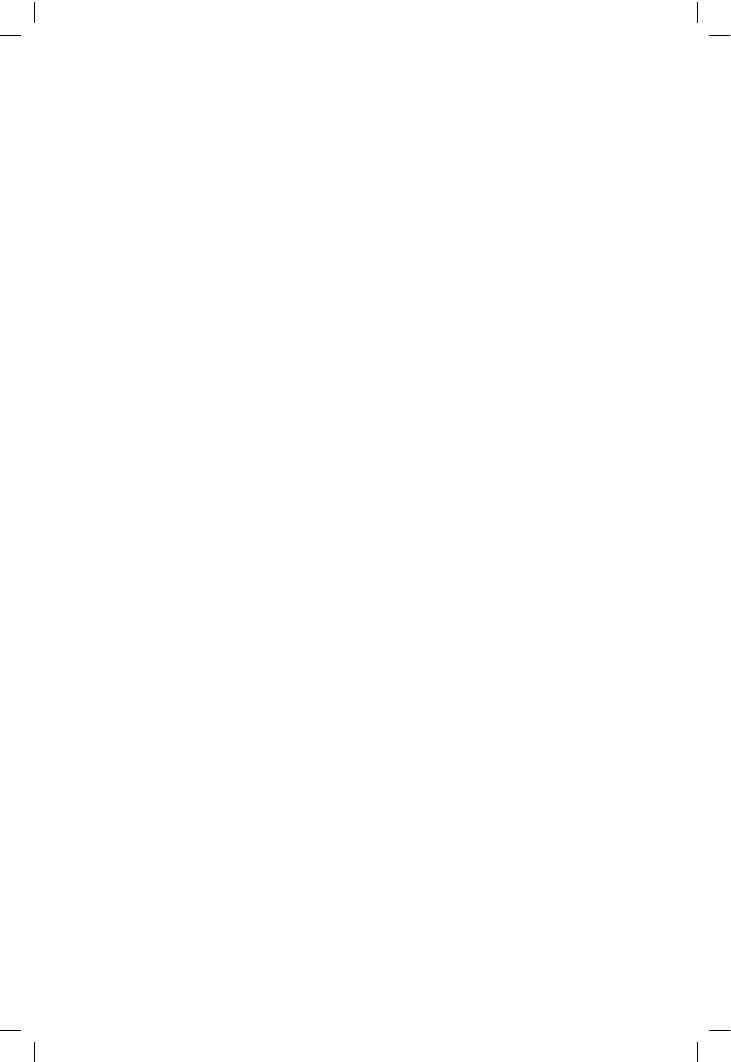
Class-A power amplifiers
The output stage is of the Complementary Feedback Pair (CFP) type,
which as previously described, gives the best linearity and quiescent
stability, due to the two local negative feedback loops around driver and
output device. Quiescent stability is particularly important with R16, 17
at 0.1 !, and this low value might be rather dicey in a double emitter-
follower (EF) output stage. The CFP voltage efficiency is also higher than
the EF version. R25, 26 define a suitable quiescent collector current for
the drivers TR6, 8, and pull charge carriers from the output device bases
when they are turning off. The lower driver is now a BD136; this has a
higher fT than the MJE350, and seems to be more immune to odd
parasitics at negative clipping.
The new lower values for the output emitter resistors R16, 17 halve the
distortion in Class-AB. This is equally effective when in Class-A with too
low a load impedance, or in Class-B but with Iq maladjusted too high. It is
now true in the latter case that too much Iq really is better than too little –
but not much better, and AB still comes a poor third in linearity to Classes
A and B.
SOAR (Safe Operating ARea) protection is given by the networks around
TR18, TR19. This is a single-slope SOAR system that is simpler than two-
slope SOAR, and therefore somewhat less efficient in terms of getting the
limiting characteristic close to the true SOAR of the output transistor. In this
application, with low rail voltages, maximum utilisation of the transistor
SOAR is not really an issue; the important thing is to observe maximum
junction temperatures in the A/AB mode.
The global negative-feedback factor is 32 dB at 20 kHz, and this should
give a good margin of safety against Nyquist-type oscillation. Global NFB
increases at 6 dB/octave with decreasing frequency to a plateau of around
64 dB, the corner being at a rather ill-defined 300 Hz; this is then
maintained down to 10 Hz. It is fortunate that magnitude and frequency
here are non-critical, as they depend on transistor beta and other doubtful
parameters.
It is often stated in hi-fi magazines that semiconductor amplifiers sound
better after hours or days of warm-up. If this is true (which it certainly isn’t
in most cases) it represents truly spectacular design incompetence. This sort
of accusation is applied with particular venom to Class-A designs, because
it is obvious that the large heatsinks required take time to reach final
temperature, so I thought it important to state that in Class-A this design
stabilises its electrical operating conditions in less than a second, giving the
full intended performance. No warm-up time beyond this is required;
obviously the heatsinks take time to reach thermal equilibrium, but as
described above, measures have been taken to ensure that component
temperature has no significant effect on operating conditions or
performance.
285
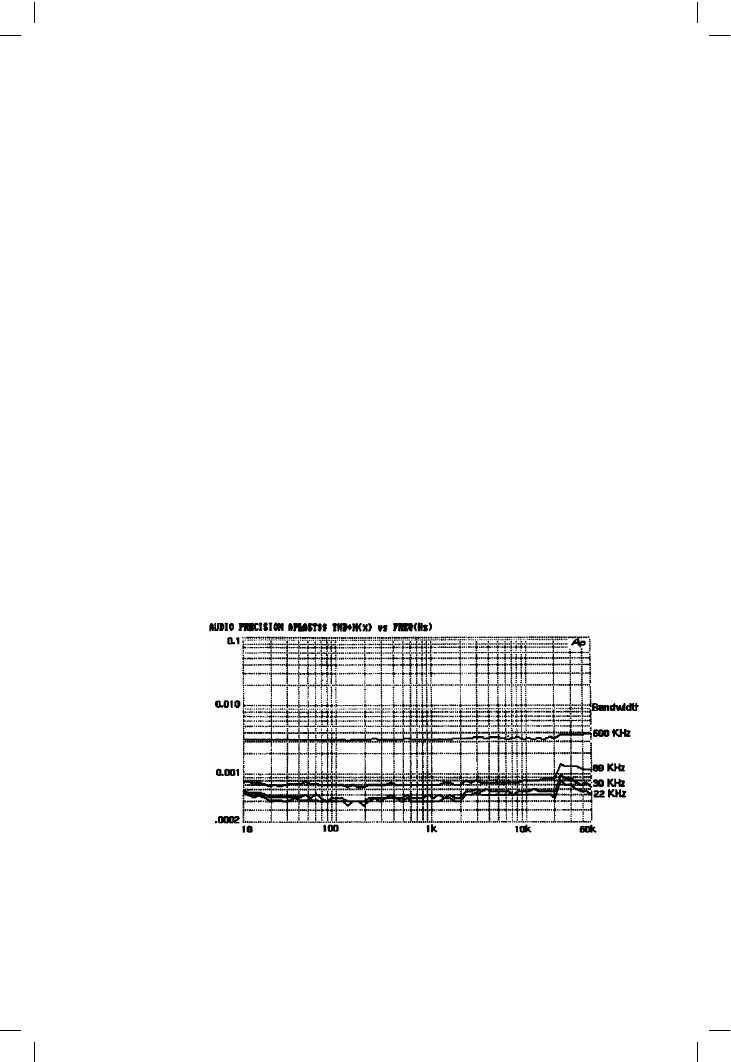
Audio Power Amplifier Design Handbook
The power supply
A suitable unregulated power supply is that shown in Figure 8.1; a
transformer secondary voltage of 20–0–20 V rms and reservoirs totalling
20,000 µF per rail will give approx. +/–24 V. This supply must be designed
for continuous operation at maximum current, so the bridge rectifier must be
properly heat-sunk, and careful consideration given to the ripple-current
ratings of the reservoirs. This is one reason why reservoir capacitance has
been doubled to 20,000 µF per rail, over the 10,000 µF that was adequate for
the Class-B design; the ripple voltage is halved, which improves voltage
efficiency as it is the ripple troughs that determine clipping onset, but in
addition the ripple current, although unchanged in total value, is now split
between two components. (The capacitance was not increased to reduce
ripple injection, which is dealt with far more efficiently and economically by
making the PSRR high.) Do not omit the secondary fuses; even in these
modern times rectifiers do fail, and transformers are horribly expensive . . .
The performance
The performance of a properly-designed Class-A amplifier challenges the
ability of even the Audio Precision measurement system. To give some
perspective on this, Figure 9.20 shows the distortion of the AP oscillator
driving the analyser section directly for various bandwidths. There appear
to be internal mode changes at 2 kHz and 20 kHz, causing step increases in
oscillator distortion content; these are just visible in the THD plots for
Class-A mode.
Figure 9.21 shows Class-B distortion for 20 W into 8 and 4 !, while Figure
9.22 shows the same in Class-A/AB. Figure 9.23 shows distortion in Class-A
for varying measurement bandwidths. The lower bandwidths misleadingly
ignore the HF distortion, but give a much clearer view of the excellent
286
Figure 9.20
The distortion in the
AP-1 system at various
measurement
bandwidths
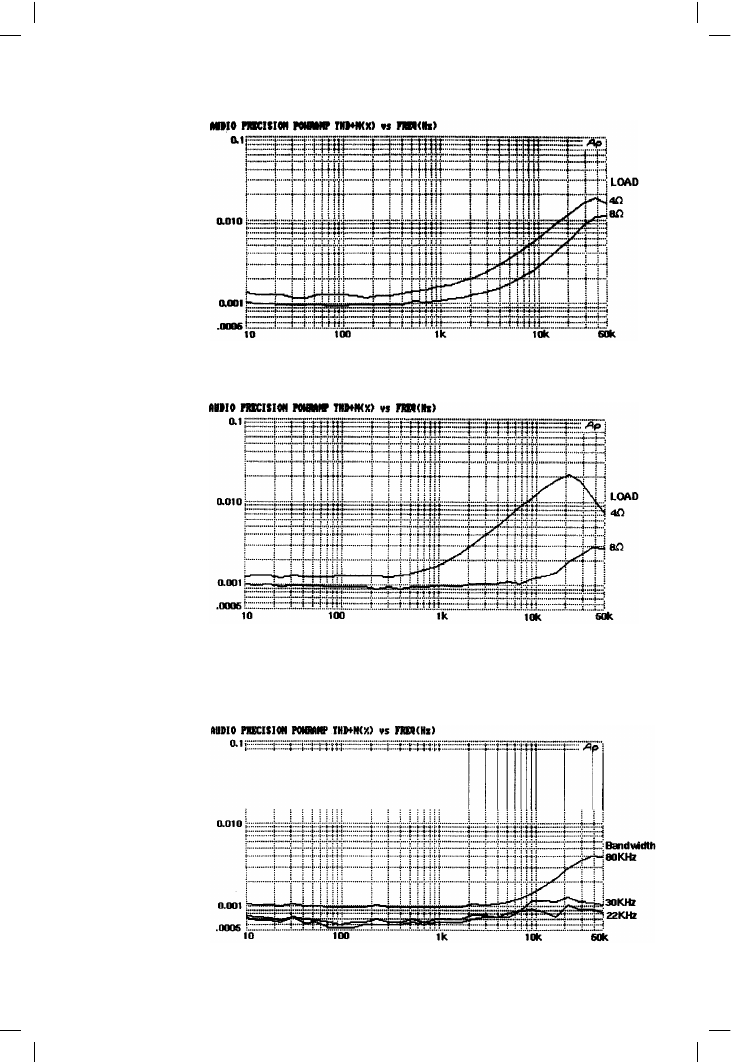
Class-A power amplifiers
287
Figure 9.21
Distortion in Class-B
(Summer) mode.
Distortion into 4 ! is
always worse. Power
was 20 W in 8 !
and 40 W in 4 !,
bandwidth 80 kHz
Figure 9.22
Distortion in Class-
A/AB (Winter) mode,
same power and
bandwidth of Figure
9.21. The amplifier is
in AB mode for the
4 ! case, and so
distortion is higher
than for Class-B into
4 !. At 80 kHz
bandwidth, the
Class-A plot below
10 kHz merely shows
the noise floor
Figure 9.23
Distortion in Class-A
only (20 W/8 !) for
varying measurement
bandwidths. The
lower bandwidths
ignore HF distortion,
but give a much
clearer view of the
excellent linearity
below 10 kHz
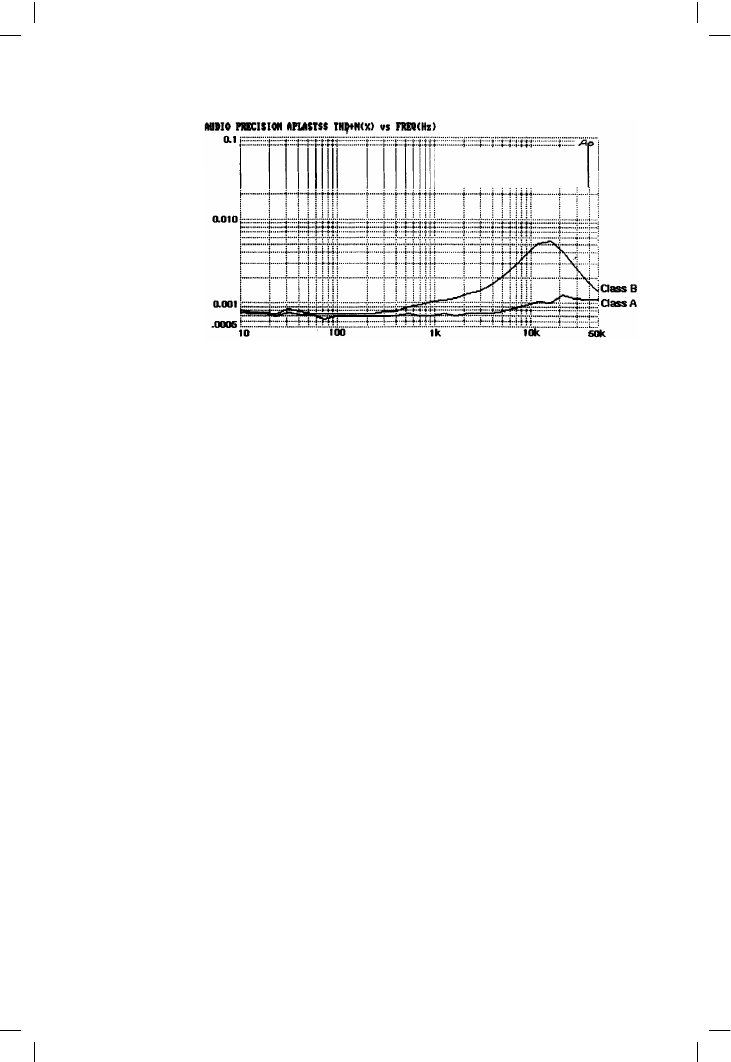
Audio Power Amplifier Design Handbook
linearity below 10 kHz. Figure 9.24 gives a direct comparison of Classes A
and B. The HF rise for B is due to high-order crossover distortion being
poorly linearised by negative feedback that falls with frequency.
Further possibilities
One interesting extension of the ideas presented here is the Adaptive
Trimodal Amplifier. This would switch into Class-B on detecting device or
heatsink overtemperature, and would be a unique example of an amplifier
that changed mode to suit the operating conditions. The thermal protection
would need to be latching; flipping from Class-A to Class-B every few
minutes would subject the output devices to unnecessary thermal
cycling.
References
1. Moore, B J An Introduction To The Psychology of Hearing Academic
Press, 1982, pp. 48–50.
2. Tanaka, S A New Biasing Circuit for Class-B Operation Journ. Audio
Eng. Soc. Jan/Feb 1981, p. 27.
3. Fuller, S Private communication.
4. Nelson Pass Build A Class-A Amplifier Audio, Feb 1977, p. 28
(Constant-current).
5. Linsley-Hood, J Simple Class-A Amplifier Wireless World, April 1969,
p. 148.
6. Self, D High-Performance Preamplifier Wireless World, Feb 1979,
p. 41.
7. Nelson-Jones, L Ultra-Low Distortion Class-A Amplifier Wireless
World, March 1970, p. 98.
288
Figure 9.24
Direct comparison of
Classes A and B
(20 W/8 !) at
30 kHz bandwidth.
The HF rise for B is
due to the inability of
negative feedback
that falls with
frequency to linearise
the high-order
crossover distortion in
the output stage
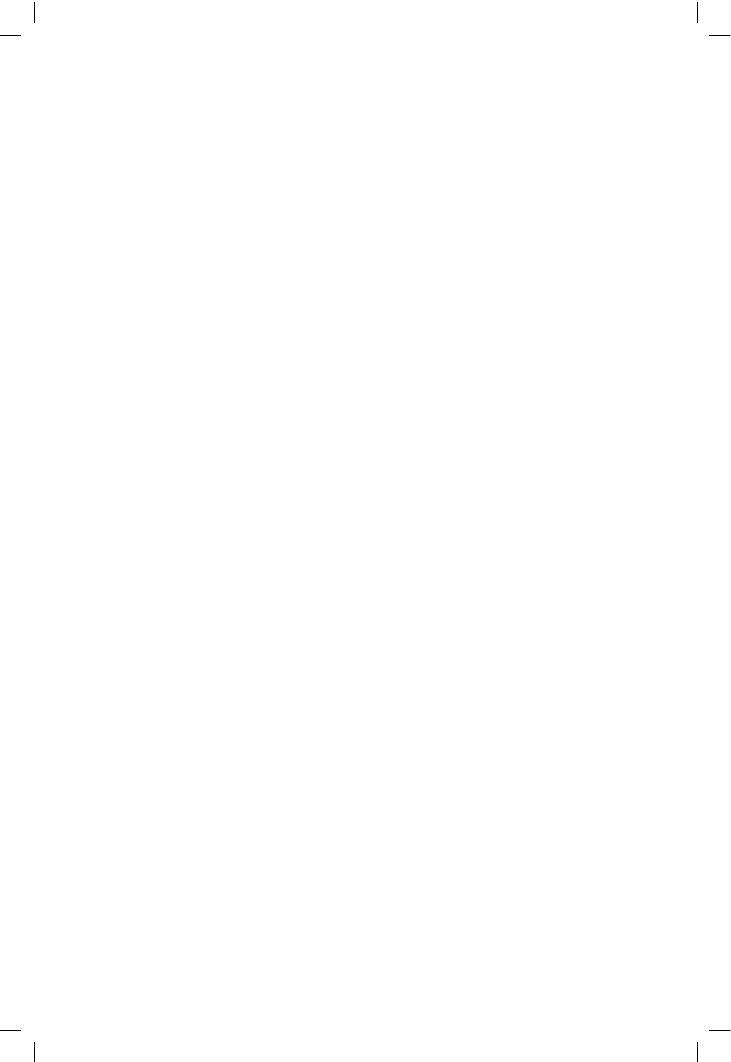
Class-A power amplifiers
8. Giffard, T Class-A Power Amplifier Elektor Nov 1991, p. 37.
9. Linsley-Hood, J High-Quality Headphone Amp HiFi News and RR, Jan
1979, p. 81.
10. Nelson Pass The Pass/A40 Power Amplifier The Audio Amateur, 1978,
p. 4 (Push-pull).
11. Thagard, N Build a 100 W Class-A Mono Amp Audio, Jan 95, p. 43.
289