ВУЗ: Казахская Национальная Академия Искусств им. Т. Жургенова
Категория: Учебное пособие
Дисциплина: Не указана
Добавлен: 03.02.2019
Просмотров: 17363
Скачиваний: 18
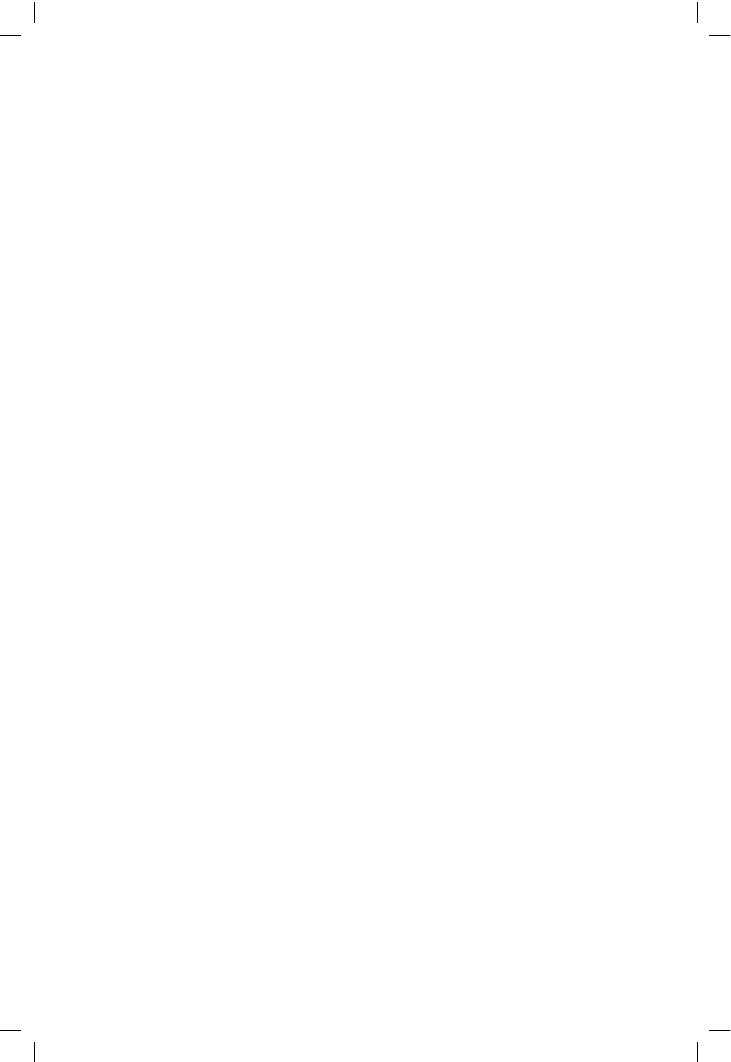
13
Amplifier and
loudspeaker protection
Categories of amplifier protection
The protection of solid-state amplifiers against overload is largely a matter
of safeguarding them from load impedances that are too low and endanger
the output devices; the most common and most severe condition being a
short across the output. This must be distinguished from the casual use of
the word overload to mean excessive signal that causes clipping and
audible distortion.
Overload protection is not the only safety precaution required. An equally
vital requirement is DC-offset protection – though here it is the loudspeaker
load that is being protected from the amplifier, rather than the other way
round.
Similarly, thermal protection is also required for a fully-equipped amplifier.
Since a well-designed product will not overheat in normal operation, this
is required to deal with two abnormal conditions:
1 The amplifier heatsinking is designed to be adequate for the reproduc-
tion of speech and music (which has a high peak-to-volume ratio, and
therefore brings about relatively small dissipation) but cannot sustain
long-term sinewave testing into the minimum specified load impedance
without excessive junction temperatures. Heatsinking forms a large part
of the cost of any amplifier, and so economics makes this a common state
of affairs.
Similar considerations apply to the rating of amplifier mains transformers,
which are often designed to indefinitely supply only 70% of the current
required for extended sinewave operation. Some form of thermal cut-out
in the transformer itself then becomes essential (see Chapter 8).
370
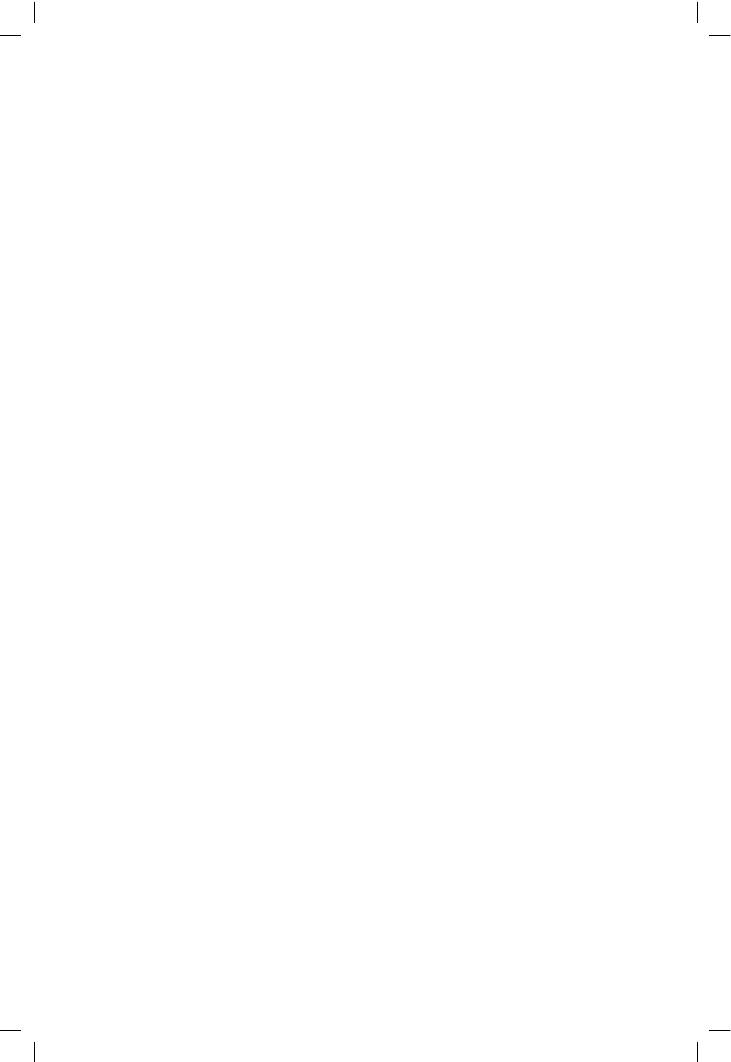
Amplifier and loudspeaker protection
2 The amplifier is designed to withstand indefinite sinewave testing, but is
vulnerable to having ventilation slots, etc. blocked, interfering either
with natural convection or fan operation.
Finally, all amplifiers require internal fusing to minimise the consequences
of a component failure – i.e. protecting the amplifier from itself – and to
maintain safety in the event of a mains wiring fault.
Semiconductor failure modes
Solid-state output devices have several main failure modes, including
excess current, excess power dissipation, and excess voltage. These are
specified in manufacturer’s data sheets as Absolute Maximum Ratings,
usually defined by some form of words such as exceeding these ratings
even momentarily may cause degradation of performance and/or reduction
in operating lifetime. For semiconductor power devices ratings are usually
plotted as a Safe Operating Area (SOA) which encloses all the permissible
combinations of voltage and current. Sometimes there are extra little areas,
notably those associated with second-breakdown in BJTs, with time limits
(usually in microseconds) on how long you can linger there before
something awful happens.
It is of course also possible to damage the base-emitter junction of a BJT by
exceeding its current or reverse voltage ratings, but this is unlikely in power
amplifier applications. In contrast the insulated gate of an FET is more
vulnerable and zener clamping of gate to source is usually considered
mandatory, especially since FET amplifiers often have separate higher
supply-rails for their small-signal sections.
BJTs have an additional important failure mode known as second
breakdown, which basically appears as a reduction in permissible power
dissipation at high voltages, due to local instability in current flow. The
details of this mechanism may be found in any textbook on transistor
physics.
Excessive current usually causes failure when the I
2
R heating in the bond
wires becomes too great and they fuse. This places a maximum on the
current-handling of the device no matter how low the voltage across it, and
hence the power dissipation. In a TO3 package only the emitter bond wire
is vulnerable, as the collector connection is made through the transistor
substrate and flange. If this wire fails with high excess current then on some
occasions the jet of vaporised metal will drill a neat hole through the top of
the TO3 can – an event which can prove utterly mystifying to those not in
the know.
Any solid-state device will fail from excess dissipation, as the internal
heating will raise the junction temperatures to levels where permanent
degradation occurs.
371
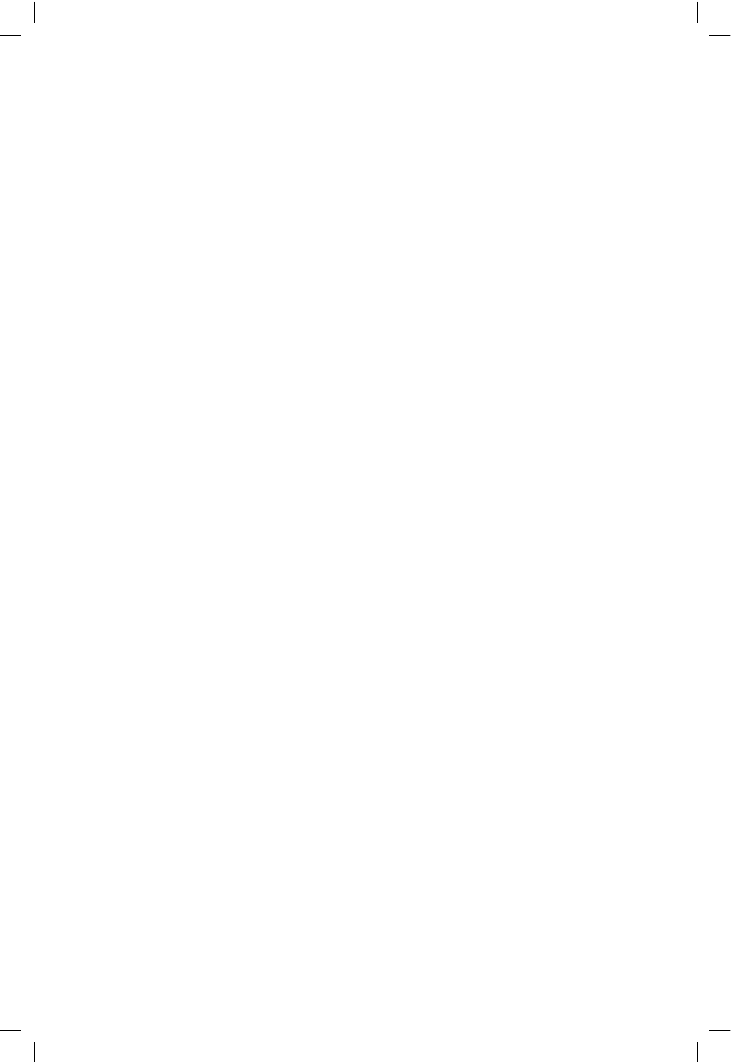
Audio Power Amplifier Design Handbook
Excess emitter-collector or source-drain voltage will also cause failure. This
failure mode does not usually require protection as such, because
designing against it should be fairly easy. With a resistive load the
maximum voltage is defined by the power supply-rails, and when the
amplifier output is hard against one rail the voltage across the device that
is turned off will be the sum of the two rails, assuming a DC-coupled
design. If devices with a Vce(max.) greater than this is selected there should
be no possibility of failure. However, practical amplifiers will be faced with
reactive load impedances, and this can double the Vce seen by the output
devices. It is therefore necessary to select a device that can withstand at
least twice the sum of the HT rail voltages, and allow for a further safety
margin on top of this. Even greater voltages may be generated by abrupt
current changes in inductive loads, and these may go outside the supply-
rail range causing device failure by reverse biasing. This possibility is
usually dealt with by the addition of catching diodes to the circuit (see
below) and does not in itself affect the output device specification
required.
Power semiconductors have another failure mode initiated by repeated
severe temperature changes. This is usually known as thermal cycling and
results from stresses set up in the silicon by the differing expansion
coefficients of the device chip and the header it is bonded to. This
constitutes the only real wearout mechanism that semiconductors are
subject to. The average lifetimes of a device subjected to temperature
variations delta–T can be approximately predicted by the equation:
N = 10
7
· e
–0.05·del–T
Equation 13.1
Where N = cycles to failure, and delta–T is the temperature change.
This shows clearly that the only way open to the designer to minimise the
risk of failure is to reduce the temperature range or the number of
temperature cycles. Reducing the junction temperature range requires
increasing heatsink size or improving the thermal coupling to it. Thermal
coupling can be quickly improved by using high-efficiency thermal
washers, assuming their increased fragility is acceptable in production, and
this is much more cost-effective than increasing the weight of heatsink. The
number of cycles can only be minimised by leaving equipment (such as
Class-A amplifiers) powered long-term, which has distinct disadvantages in
terms of energy consumption and possibly safety.
Overload protection
Solid-state output devices are much less tolerant to overload conditions
than valves, and often fail virtually instantaneously. Some failure modes
(such as overheating) take place slowly enough for human intervention, but
this can never be relied upon. Overload protection is therefore always an
important issue, except for specialised applications such as amplifiers built
372
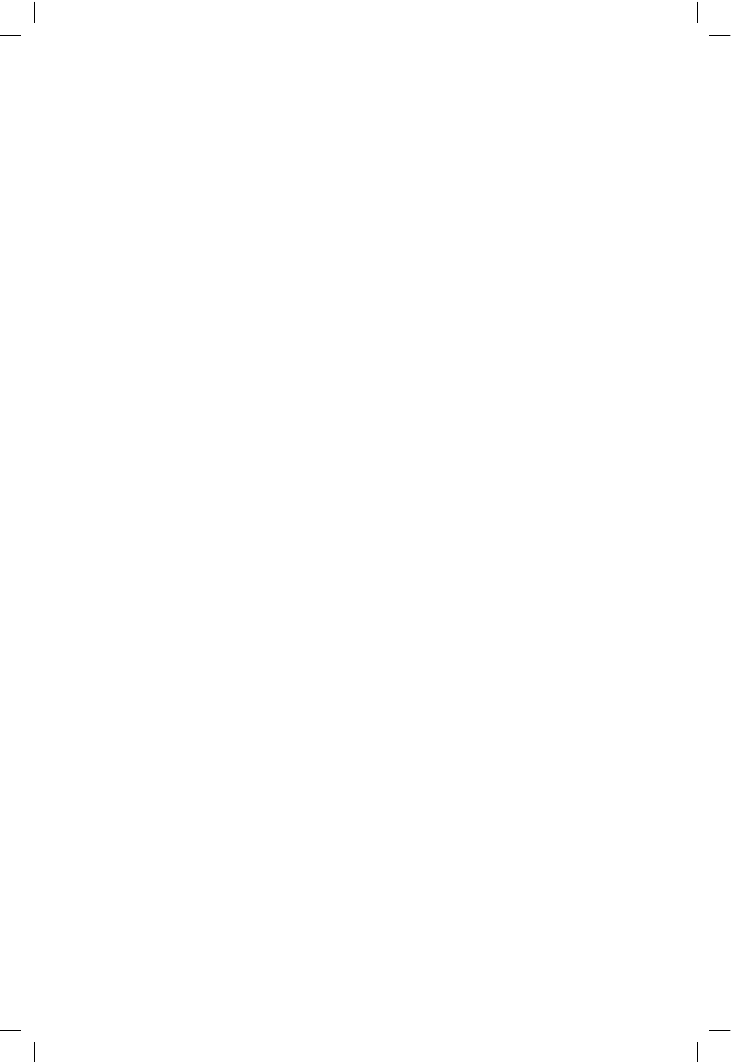
Amplifier and loudspeaker protection
into powered loudspeakers, where there are no external connections and
no possibility of inadvertent short-circuits.
Driven by necessity, workable protection systems were devised relatively
early in the history of solid-state amplifiers; see Bailey
[1]
, Becker
[2]
and
Motorola
[3]
. Part of the problem is defining what constitutes adequate
current delivery into a load. Otala
[4]
has shown that a complex impedance,
i.e. containing energy-storage elements, can be made to draw surprisingly
large currents if specially optimised pulse waveforms are used that catch
the load at the worst part of the cycle; however it seems to be the general
view that such waveforms rarely if ever occur in real life.
Verifying that overload protection works as intended over the wide range of
voltages, currents, and load impedances possible is not a light task. Peter
Baxandall introduced a most ingenious method of causing an amplifier to
plot its own limiting lines
[5]
.
Overload protection by fuses
The use of fuses in series with the output line for overload protection is no
longer considered acceptable, as it is virtually impossible to design a fuse
that will blow fast enough to protect a semiconductor device, and yet be
sufficiently resistant to transients and turn-on surges. There are also the
obvious objections that the fuse must be replaced every time the protection
is brought into action, and there is every chance it will be replaced by a
higher value fuse which will leave the amplifier completely vulnerable.
Fuses can react only to the current flowing through them, and are unable
to take account of other important factors such as the voltage drop across
the device protected.
Series output fuses are sometimes advocated as a cheap means of DC offset
protection, but they are not dependable in this role.
Putting a fuse in series with the output will cause low-frequency distortion
due to cyclic thermal changes in the fuse resistance. The distortion problem
can, in theory at least, be side-stepped by putting the fuse inside the global
feedback loop; however what will the amplifier do when its feedback is
abruptly removed when the fuse blows? (See also page 384 on DC offset
protection below.)
One way of so enclosing fuses that I have seen advocated is to use them
instead of output emitter-resistors Re; I have no personal experience of this
technique, but since it appears to add extra time-dependent thermal
uncertainties (due to the exact fuse resistance being dependant upon its
immediate thermal history) to a part of the amplifier where they already
cause major difficulties, I don’t see this as a promising path to take. There
is the major difficulty that the failure of only one fuse will generate a
maximal DC offset, so we may have dealt with the overload, but there is
373
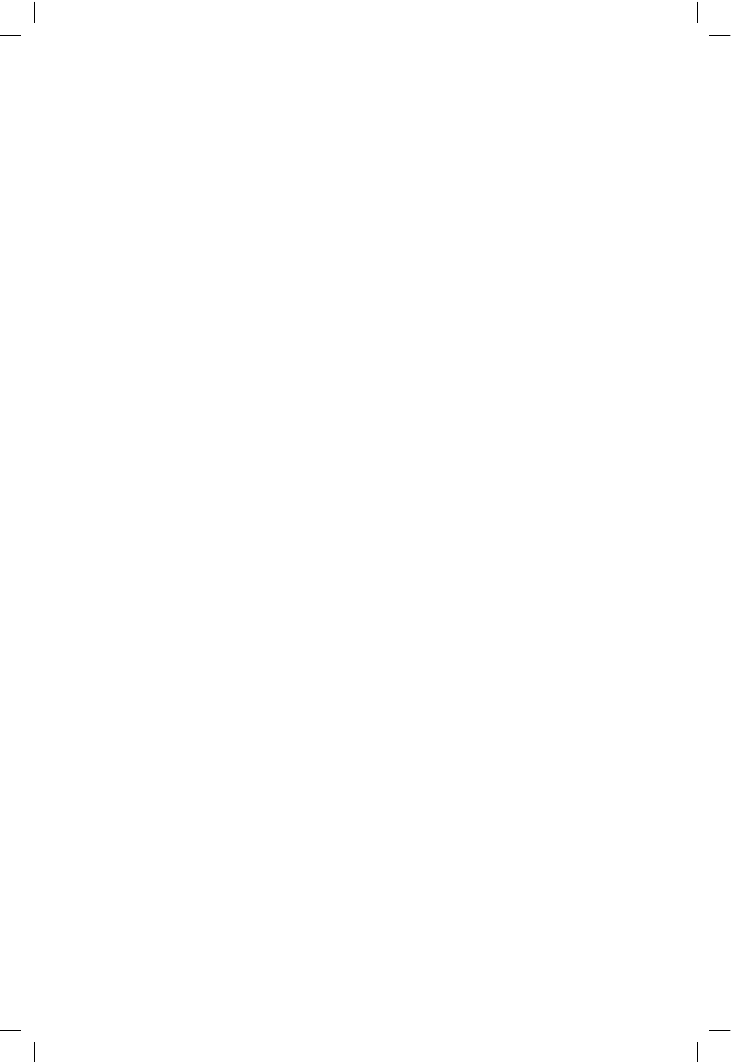
Audio Power Amplifier Design Handbook
now a major DC offset to protect the loudspeaker from. The other fuse may
blow as a consequence of the large DC current flow, but sizing a fuse to
protect properly against both overload and DC offset may prove
impossible.
Amplifier circuitry should always include fuses in each HT line. These are
not intended to protect the output devices, but to minimise the damage when
the output devices have already failed. They can and should therefore be of
the slow-blow type, and rated with a good safety margin, so that they are
entirely reliable; a fuse operated anywhere near its nominal fusing current
has a short life-time, due to heating and oxidation of the fuse wire. HT fuses
cannot save the output devices, but they do protect the HT wiring and the
bridge rectifier, and prevent fire. There should be separate DC fuses for each
channel, as this gives better protection than one fuse of twice the size, and
allows one channel to keep working in an emergency.
Similarly, the mains transformer secondaries should also be fused. If this is
omitted, a failure of the rectifier will inevitably cause the mains transformer
to burn out, and this could produce a safety hazard. The secondary fuses
can be very conservatively rated to make them reliable, as the mains
transformer should be able to withstand a very large fault current for a short
time. The fuses must be of the slow-blow type to withstand the current
surge into the reservoir capacitors at switch-on.
The final fuse to consider is the mains fuse. The two functions of this are to
disconnect the live line if it becomes shorted to chassis, and to protect
against gross faults such as a short between live and neutral. This fuse must
also be of the slow-blow type, to cope with the transformer turn-on current
surge as well as charging the reservoirs. In the UK, there will be an
additional fuse in the moulded mains plug. This does not apply to mains
connectors in other countries and so a mains fuse built into the amplifier
itself is absolutely essential.
Electronic overload protection
There are various approaches possible to overload protection. The
commonest form (called electronic protection here to distinguish it from
fuse methods) uses transistors to detect the current and voltage conditions
in the output devices, and shunts away the base drive from the latter when
the conditions become excessive. This is cheap and easy to implement (at
least in principle) and since it is essentially a clamping method requires no
resetting. Normal output is resumed as soon as the fault conditions are
removed. The disadvantage is that a protection scheme that makes good
use of the device Safe Operating Area (SOA) may allow substantial
dissipation for as long as the fault persists undetected, and while this should
not cause short-term failure if the protection has been correctly designed,
the high temperatures generated may impair long-term reliability.
374