Файл: Курсовая работа по дисциплине Процессы и аппараты химической технологии на тему Adsorption.docx
Добавлен: 07.11.2023
Просмотров: 88
Скачиваний: 3
ВНИМАНИЕ! Если данный файл нарушает Ваши авторские права, то обязательно сообщите нам.
СОДЕРЖАНИЕ
2.2 Properties and Physical characteristics of adsorbents.
3 APPLICATIONS OF ADSORPTION PROCESS
4.1 Introduction to adsorption processes in water treatment
4.2 Adsorption in drinking water treatment
4.3 Adsorption in wastewater treatment
4.4 Adsorption in hybrid processes in water treatment
5 ADSORBENTS FOR WATER TREATMENT
5.1 Introduction to adsorbent classification for water treatment
5.2 Activated carbon as an engineered adsorbent
5.3 Polymeric adsorbents as an engineered adsorbent
5.4 Oxidic adsorbents as engineered adsorbents
5.5 Synthetic zeolites as engineered adsorbents
Adsorbents used in drinking water treatment must meet strict quality requirements and are frequently required to be certified in order to ensure the safety of the water. As a result, there are only a few potential adsorbents, primarily commercial activated carbons and oxidic adsorbents. The LCAs and other adsorbents work well for the treatment of wastewater. [17]
Given that adsorption is a surface process, the adsorbent's surface area plays a crucial role in determining the extent of adsorption and is thus an important quality parameter. The surface areas of naturally occurring adsorbents are typically much smaller than those of highly porous engineered adsorbents. The largest surface areas can be found in special polymeric adsorbents and activated carbons. High porosity of the material is a requirement for high surface area because it makes it possible for the pore walls to form a significant portion of the material's internal surface. [15]
Engineered adsorbents have internal surfaces that are significantly larger than their particle surfaces on the outside. The internal surface is typically higher when the pore system is larger and the pores are smaller. On the other hand, to enable quick adsorbate transport to the adsorption sites, a certain fraction of larger pores is required. Consequently, pore-size distribution is yet another crucial quality factor. The surface chemistry, in addition to the texture, may also be of interest, particularly for chemisorption processes. [16]
5.2 Activated carbon as an engineered adsorbent
Since ancient times, carbon-reach materials' adsorption capacities have been understood; however, it wasn't until the first decades of the 20th century that these materials' adsorption capacities were enhanced by unique activation processes. Different carbon-containing raw materials and activation techniques can be used to create various types of activated carbons. Wood, wood charcoal, peat, lignite and lignite coke, hard coal and coke, bituminous coal, petrol coke, and residual materials like coconut shells, sawdust, or plastic residues are the most typical raw materials. [18]
For organic raw materials like wood, sawdust, peat, or coconut shells, a preliminary carbonization process is necessary to transform the cellulose structures into a carbonaceous material. Such cellulose structures contain a number of oxygen- and hydrogen-containing functional groups, which can be removed by dehydrating chemicals. The dehydration is typically carried out at elevated temperatures under pyrolytic conditions and leads to a destruction of the cellulose structures with the result that the carbon skeleton is left. This process, referred to as chemical activation, combines carbonization and activation processes. Typical dehydrating chemicals are zinc chloride and phosphoric acid. After cooling the product, the activation agent has to be extracted. Since the extraction is often not complete, residuals of the activation chemicals remain in the activated carbon and might be leached during the application. This is in particular critical for drinking water treatment. Furthermore, the application of chemicals in the activation process requires an expensive recycling, and the products of chemical activation are typically powders with low densities and low content of micropores. For these reasons, most of the activated carbons used in drinking water treatment are produced by an alternative process termed physical, thermal, or gas activation. [20]
In gas activation, carbonized materials such as coals or cokes are used as raw materials. These carbon-rich materials already have certain porosity. For activation, the raw material is brought in contact with an activation gas (steam, carbon dioxide, air) at elevated temperatures (800˚C–1,000˚C). During the activation, the activation gas reacts with the solid carbon to form gaseous products. In this manner, closed pores are opened and existing pores are enlarged. The reactions cause a mass loss of the solid material. Since the development of the pore system and the surface area are correlated with the burn-off, an optimum for the extent of the activation has to be found. This optimum depends on the material and is often in the range of 40 % to 50 % burn-off. Higher burn-off degrees lead to a decrease of net surface area because no more new pores are opened, but existing pore walls are burned away. [19]
The reactions in gas activation are the same as in the carbo-chemical process of coal gasification for synthesis gas production, but in contrast to it, the gasification is not complete. A positive sign of the reaction enthalpy indicates an endothermic process, whereas a negative sign indicates an exothermic process. The products of gas activation mainly occur in granulated form. Different particle sizes can be obtained by grinding and sieving. Gas activation processes can also be used for a further activation of chemically activated carbons. [18]
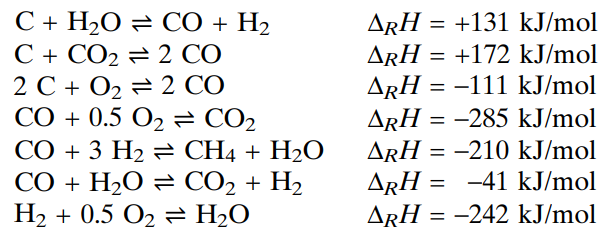
Activated carbons are applied in two different forms, as granular activated carbon (GAC) with particle sizes in the range of 0.5 to 4 mm and powdered activated carbon (PAC) with particle sizes < 40 μm. The different particle sizes are related to different application techniques: slurry reactors for PAC (powdered activated carbon) application and fixed-bed adsorbers for GAC (granulated activated carbon). Activated carbons show a broad variety of internal surface areas ranging from some hundreds m2 /g to more than a thousand m2 /g depending on the raw material and the activation process used. Activated carbon for water treatment should not have pores that are too fine so that larger molecules are also allowed to enter the pore system and to adsorb onto the inner surface. Internal surface areas of activated carbons applied for water treatment are typically in the range of 800–1000 m
2 /g. [19]
The activated carbon structure consists of crystallites with a strongly disturbed graphite structure. In graphite, the carbon atoms are located in layers and are connected by covalent bonds. Graphite possesses a delocalized π-electron system that is able to interact with aromatic structures in the adsorbate molecules. The graphite crystallites in activated carbon are randomly oriented and interconnected by carbon cross-links. The micropores are formed by the voids between the crystallites and are therefore typically of irregular shape. Frequently, slit-like pores are found. [17]
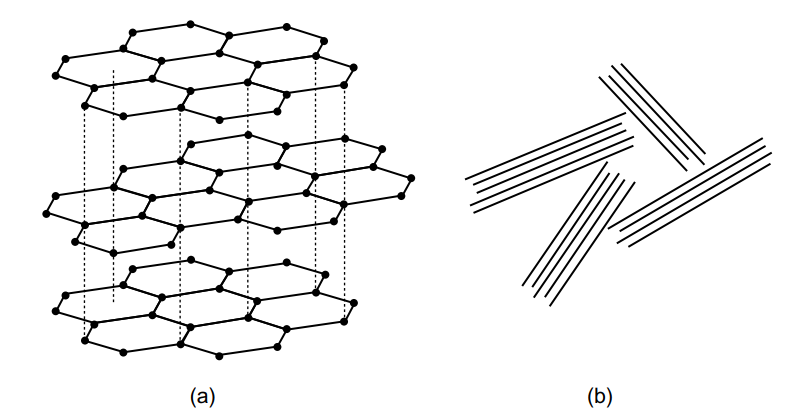
Figure 5.1 Structural elements of activated carbons:
a - graphite structure; b - randomly oriented graphite micro-crystallites
Loaded activated carbon is typically regenerated by thermal processes. In most cases, the activated carbon is reactivated analogously to the gas activation process. Reactivation causes a mass loss due to burn-off. PAC cannot be reactivated and is therefore used as a one-way adsorbent and has to be burned or deposited after application. [19]
5.3 Polymeric adsorbents as an engineered adsorbent
Polymeric adsorbents, also referred to as adsorbent resins, are porous solids with considerable surface areas and distinctive adsorption capacities for organic molecules. They are produced by copolymerization of styrene, or sometimes also acrylic acid esters, with divinylbenzene as a cross-linking agent. Their structure is comparable to that of ion exchangers, but in contrast to ion exchangers, the adsorbent resins have no or only few functional groups and are nonpolar or only weakly polar. To obtain a high porosity, the polymerization is carried out in the presence of an inert medium that is miscible with the monomer and does not strongly influence the chain growth. After polymerization, the inert medium is removed from the polymerizate by extraction or evaporation. Polymeric adsorbent materials tailored for particular needs can be produced by variation of the type and the concentration of the inert compound, the monomer concentration, the fraction of divinylbenzene, the concentration of polar monomers, and the reaction conditions. [20]
The conventional polymeric adsorbents have surface areas up to 800 m2 /g. The polymer adsorbents typically show a narrow pore-size distribution, and the surface is relatively homogeneous. With increasing degree of cross-linking, the pore size becomes smaller and the surface area increases. Below you can see the typical structure of a styrene-divinylbenzene copolymer. [19]
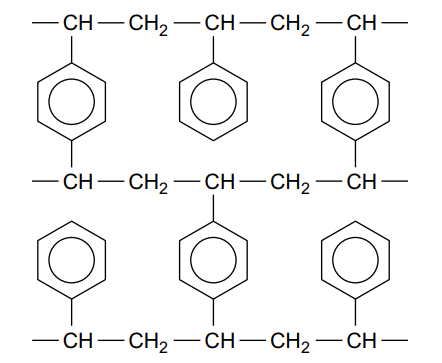
Figure 5.2 Structure of a styrene-divinylbenzene copolymer
By specific post-cross-linking reactions, such as chloromethylation with subsequent dehydrochlorination, the pore size can be further reduced and large surface areas, up to 1200 m2 /g and more, can be received. [19]
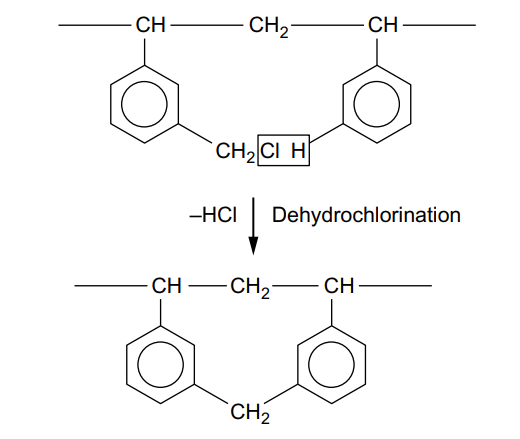
Figure 5.3 Principle of post-cross-linking of polymeric networks by chloromethylation and subsequent dehydrochlorination
Highly cross-linked polymeric adsorbents show adsorption capacities that are comparable to that of activated carbons. Desorption of the adsorbed organic compounds is possible by extraction with solvents, in particular alcohols, such as methanol or isopropanol. The much higher costs for the polymeric materials in comparison to activated carbons and the need for extractive regeneration by solvents make the polymeric adsorbents unsuitable for treatment of large amounts of water with complex composition – for instance, for drinking water treatment or treatment of municipal wastewater effluents. Instead of that, polymeric adsorbents can be beneficially applied for recycling of valuable chemicals from process wastewaters. To separate the solvent from the desorbed compounds, an additional process step – for instance, distillation – is necessary. [20]
5.4 Oxidic adsorbents as engineered adsorbents
The term of oxidic adsorbents comprises solid hydroxides, hydrated oxides, and oxides. Among the engineered oxidic adsorbents, aluminum and iron materials are the most important. The general production process is based on the precipitation of hydroxides followed by a partial dehydration at elevated temperatures. The hydroxide products are thermodynamically metastable. Further strong heating would result in a transformation to stable oxides with only small surface areas. The dehydration process of a trivalent metal (Me) hydroxide can be described in a simplified manner as:
Me(OH)3 MeO(OH) + H2O
2 MeO(OH) Me2O3 + H2O
In these reactions, species with different water contents can occur as intermediates. [17]
The oxidic adsorbents exhibit a relatively large number of surfaces OH groups, which substantially determine their adsorption properties. The polar character of the surface together with possible protonation or deprotonation processes of the OH groups make the oxidic adsorbents ideally suited for the removal of ionic compounds, such as phosphate, arsenate, fluoride, or heavy metal species. [16]
Activated aluminum oxide (γ-aluminum oxide, γ-Al2O3) can be used for the removal of arsenate and fluoride from drinking water or for the removal of phosphate from wastewater. The surface areas are in the range of 150–350 m2 /g. Activated aluminum oxide is produced in different particle sizes, ranging from about 0.1 to 10 mm. [18]
Recently, iron (III) hydroxide (ferric hydroxide) in granulated form finds increasing interest, in particular as an efficient adsorbent for arsenate, but also for phosphate and other ions. Different products are available with crystal structures according to α-FeOOH (goethite) and β-FeOOH (akageneite). The surface areas are comparable to that found for aluminum oxide and range from 150 to 350 m2 /g. Typical particle sizes are between 0.3 and 3 mm. [20]