Файл: Курсовая работа по дисциплине Процессы и аппараты химической технологии на тему Adsorption.docx
Добавлен: 07.11.2023
Просмотров: 89
Скачиваний: 3
ВНИМАНИЕ! Если данный файл нарушает Ваши авторские права, то обязательно сообщите нам.
СОДЕРЖАНИЕ
2.2 Properties and Physical characteristics of adsorbents.
3 APPLICATIONS OF ADSORPTION PROCESS
4.1 Introduction to adsorption processes in water treatment
4.2 Adsorption in drinking water treatment
4.3 Adsorption in wastewater treatment
4.4 Adsorption in hybrid processes in water treatment
5 ADSORBENTS FOR WATER TREATMENT
5.1 Introduction to adsorbent classification for water treatment
5.2 Activated carbon as an engineered adsorbent
5.3 Polymeric adsorbents as an engineered adsorbent
5.4 Oxidic adsorbents as engineered adsorbents
5.5 Synthetic zeolites as engineered adsorbents
The removal of submicron colloidal particles from fluid to solid surfaces may be described as either filtration or deposition. Carbon columns used to remove dissolved organic solutes in water treatment are often referred to as ‘carbon filters’ by water engineers. Similarly, the term ‘charcoal filter’ is used to denote cartridges filled with granular activated carbon for personal protection. In spite of these similarities, the analogy between deep-bed filtration and fixed-bed adsorption is limited.
A major difference between them resides in the fact that in deepbed filtration, removal of particles from the suspension to be treated results in particle deposition over the exterior surfaces of the filter media. In contrast, the adsorbed dissolved species in fixed-bed adsorption covers mainly the interior surfaces of adsorbents. As stated before, the extent of separation achieved in adsorption is limited by the adsorption equilibrium relationship.
On the other hand, particle retention in deep-bed filtration depends strongly upon the nature of particle-collector interaction forces, but there is no clear-cut limit on the extent of deposition. As adsorption processes may cease operation once the adsorbents become saturated, for deep-bed filtration, due to increasing particle retention, the pressure drops required for maintaining a specified throughput increase with time. The duration of operation is limited by the maximum allowable pressure drop. [1]
-
Operation modes of adsorption processes
Separation by adsorption is effected by contacting solutions to be treated with selected adsorbents. There are numerous ways of bringing about fluid/solid contact, as shown in Fig. 1.1 A brief description of is given as follows:
a. Adsorption in agitated vessels. Batch adsorption in agitated vessel represents perhaps the simplest way of bringing about fluid/adsorbent contact. A fixed amount of adsorbent of a known state is added to a volume of solution of a known solute concentration in a closed vessel. Agitation is provided by rotating stirrers in order to insure that adsorbent particles are fully suspended, and the adsorbate concentration is kept uniform throughout the solution. The data collected are the temporal evolution of the solute concentration of the solution. While batch operation is not suitable for treating large volumes of solution (such as water supplies), in general, batch adsorption test data are often used in characterizing new adsorbents for applications. [3]
b. Adsorption in continuous-flow tanks. This type of operation is often used in waste water treatment. Adsorbents in powdered form, such as activated carbon, are added directly to a particular step of a treatment process (biological or physio-chemical) for the purpose of removing a particular species of contaminant. [1]
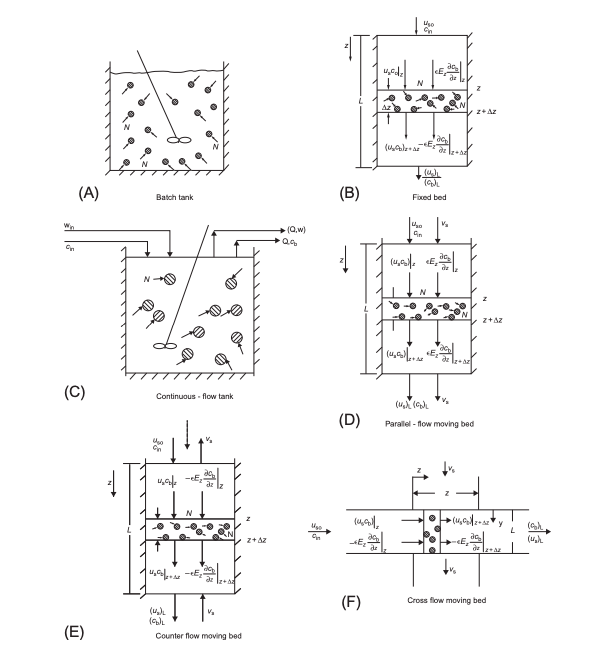
Figure 1.1 Different configurations of the adsorption process.
(a) – batch tank; (b) – fixed bed
c. Fixed-bed adsorption. Fixed-bed adsorption consists of passing a solution through a column packed with adsorbents, and is commonly applied for eliminating trace contaminants from a liquid solution, or toxic and volatile vapors from gas streams. Fixed-bed adsorption, by nature, is a batch process. Starting with a fresh or newly regenerated state, adsorbents in fixed-bed operations become increasingly saturated with adsorbate to a point that reactivation (desorption) becomes necessary, and operation ceases. However, by employing a number of identical columns and arranging the adsorption/reactivation sequence properly, a continuous operation may be established. This, in fact, is the principle for the development of pressure swing and thermal swing processes for gas separation. [1]
d. Moving-bed adsorption. In contrast to fixed-bed adsorption, moving-bed adsorption is carried out with both solid (adsorbent) and fluid phases in motion. The movements of both phases may be parallel, counter-current, or perpendicular (cross-flow); although the counter-current pattern is used in practice. [1]
2 ADSORBENTS
2.1 Adsorbent materials
To be technically effective in a commercial separation process, whether this be a bulk separation or a purification, an adsorbent material must have a high internal volume which is accessible to the components being removed from the fluid.
Such a highly porous solid may be carbonaceous or inorganic in nature, synthetic or naturally occurring, and in certain circumstances may have true molecular sieving properties. [2]
The adsorbent must also have good mechanical properties such as strength and resistance to attrition and it must have good kinetic properties, that is, it must be capable of transferring adsorbing molecules rapidly to the adsorption sites. In most applications the adsorbent must be regenerated after use and therefore it is desirable that regeneration can be carried out efficiently and without damage to mechanical and adsorptive properties. [8]
The raw materials and methods for producing adsorbents must ultimately be inexpensive for adsorption to compete successfully on economic grounds with alternative separation processes.
Most solid substances possess some adsorption capacity for gas and/or liquid, but only a few of them meet the necessary requirements to qualify as adsorbents for practical use. Some of the most widely used adsorbents are:
a. Activated carbon. Activated carbon may be produced from carbonization of materials such as coconut shells, coal, lignite, wood, and similar substances, followed by activation with air or steam. It is used widely for recovery of organic vapor, decoloring liquid solutions, and treatment and purification of water supplies and waste water.[2]
b. Activated alumina. Al2O3 is prepared by removing water from colloid alumina. It has a high capacity for water vapor, and is used mainly as a desiccant for gasses and liquids. Activated alumina can be reactivated for reuse.[5]
c. Activated silica. Activated silica is prepared from gel precipitated by acid treatment of a sodium silicate solution. Activated silica is used primarily for dehydration of air and removing toxic species from air for personal protection purposes (i.e., in gas masks).[5]
d. Molecular sieve zeolites. Most adsorbents, including those mentioned herein, have pores covering a range of sizes. Molecular sieve zeolites, however, are an exception. Molecular sieve zeolites are crystalline inorganic polymers of aluminosilicate, alkali, or alkali-earth elements such as Na, K, and Ca. The “cages” of the crystal cells can entrap adsorbed matter, and the diameter of the passageways, controlled by the crystal composition, regulate the size of the molecules that can enter. These “size-selection” characteristics differentiate molecular sieve zeolites from other adsorbents and make molecular sieve zeolites particularly useful in the separation of hydrocarbon mixtures.
e. Polymeric adsorbents. These adsorbents are prepared from polymerizing styrene and divinyl benzene or acrylic esters for adsorbing nonpolar organics from aqueous solutions or polar solutes, respectively. Generally speaking, polymeric adsorbents are more expensive than other adsorbents, and their use is, therefore, more selective.[4]
adsorbent materials have been developed recently, or are currently under development.
The structural adsorbents are a class of adsorbents fabricated in forms of simple ordered geometry, such as monolith, laminate, or foam. Such adsorbents provide simple, identifiable flow channels, and offer low resistance to fluid flow. Compared with granular adsorbents, they also offer low resistance to mass transfer. These adsorbents are, therefore, of particular interest for applications in pressure swing adsorption. Similar to commonly used zeolites, metal organic frameworks (MOFs) and covalent organic frameworks (COFs) are porous crystalline solids. The metal organic frameworks are compounds consisting of metal ions or clusters coordinated to organic ligands to form one-, two-, and three-dimensional structures. Covalent organic frameworks are a new type of organic material constructed with organic building units via covalent bonds. This well-defined crystalline porous structure, together with tailored functionalities, are known for their unique features. Both MOFs and COFs supposedly have great potential for gas storage, gas adsorption, and as catalysts as well as sensors. [2]
2.2 Properties and Physical characteristics of adsorbents.
The morphological features of the adsorbent constitute the physical characteristics such as surface area, particle size, pore size, and density. The porosity of any solid is the part of the space available in the total volume of the material. Generally, the more porous the material, the more will be its adsorption capacity.
The external surface area is supposed to influence the mass transfer rate during the adsorption process. For porous adsorbents, the external mass transfer occurs via the formation of hydrodynamic sheath around the adsorbent, whereas internal mass transfer happens by way of intra-particle diffusion processes. The internal surface area is considered as the most important parameter of any adsorbent as the adsorption capacity of the adsorbents totally depends upon it. Sometimes, it exceeds several fold the external surface area of the porous adsorbents. This is the reason that led research groups worldwide to give emphasis on the synthesis of materials with extremely large internal surface areas.[2]
The materials possessing large internal surface areas are endowed with a plethora of pores of variable shapes and sizes that form the basis of their classification into macropores, mesopores, and micropores based on their sizes. Macropores and mesopores play a significant role in the mass transfer of adsorbate into the internal part of the adsorbent, and the volume possessed by the micropores aids in the determination of size of the internal surface and, in turn, the adsorption capacity of the adsorbent. Higher micropore volume exhibits the larger quantity of the material that will be adsorbed. However, in the case of minute pores and large adsorbate molecules, the size exclusion limits the extent of adsorption.[2]
The particle size is of extreme importance in the case where nanomaterials are used as adsorbents. The unique features that endow them as ideal adsorbents are all size-dependent, particularly large surface area and surface area-to-volume ratio leading to their improved adsorption capacity. The high surface area provides a greater number of active sites for interaction with a diverse class of contaminants. The particles having their sizes in the nanoscale range are highly reactive and possess catalytic potential, enabling them as a better alternative than conventional materials.[5]
The interaction between the adsorbate and the adsorbent is largely affected by the surface chemistry of the adsorbent, especially for the case where adsorption of ions occurs onto oxidic surfaces (Worch 2012a). In this perspective, the pHpzc of the adsorbent assists in the comprehension of the influence of pH on the overall adsorption of charged species on their surfaces. The point of zero charge (pHpzc) evaluates the point at which any adsorbent is electrically neutral in nature and is referred to as a region in the pH scale where the surface bears a net zero charge due to the equal sum of positive and negative charges on the surface of the adsorbent. When the pH of the medium is lower than the pHpzc of the adsorbent - the surface becomes positively charged and the medium becomes acidic due to donation of protons more than the hydroxide groups by the acidic water (Roushani et al. 2017). Thus, attraction of negatively charged species becomes feasible toward positive adsorbent surface. On the contrary, at a pH value higher than the pHpzc, the surface bears negative charges elicited by the attraction of cations and vice versa. The point of zero charge (pHpzc) is the characteristic feature of adsorbents. Generally, the electrostatic attraction/repulsion forces strongly affect the adsorption of charged adsorbate molecules on the charged adsorbent surfaces.[9]
In recent years, considerable effort has been made in the modification of adsorbent surfaces or functionalization of adsorbents in order to overcome their inherent limitations and enhance their low adsorption capacities and selectivity of both organic and inorganic moieties from water. The adsorbent surfaces are being modified with a variety of different compounds to alter their wide range of surface characteristics such as stability, functionality, reactivity, biocompatibility, dispensability, and inertness in intolerable environments (Manyangadze et al. 2020). Surface modification or functionalization has been carried out with a multiplicity of agents; for example, some carbon materials are activated by H
2SO4, H,PO4, ZnCl2, H2O2, KOH, etc., and certain ligands are also used, for example, surfactants, polymers, dendrimers, small molecules, and biomolecules. Surface modification also aids in controlling the aggregation of nanoparticles, which is their perennial property, assists in their easy separation when the surface is magnetically functionalized, results in high density of reactive sites, and assures high and rapid adsorption rates. Oftentimes, it is witnessed that the degree of modification/functionalization of adsorbent surface leads to a decrease in specific surface area but eventually results in an increased adsorption capacity.[10]