ВУЗ: Казахская Национальная Академия Искусств им. Т. Жургенова
Категория: Учебное пособие
Дисциплина: Не указана
Добавлен: 03.02.2019
Просмотров: 17370
Скачиваний: 18
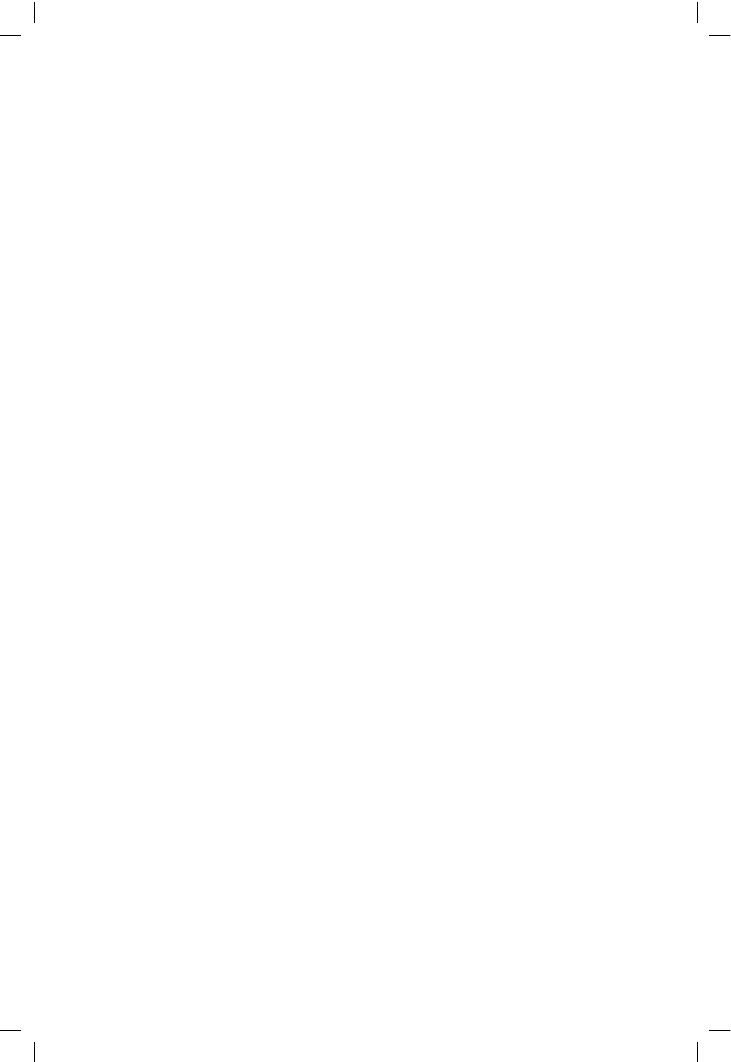
3
The general principles of
power amplifiers
How a generic amplifier works
Figure 3.1 shows a very conventional power amplifier circuit; it is as
standard as possible. A great deal has been written about this configuration,
though the subtlety and quiet effectiveness of the topology are usually
overlooked, and the explanation below therefore touches on several
aspects that seem to be almost unknown. The circuit has the merit of being
docile enough to be made into a functioning amplifier by someone who
has only the sketchiest of notions as to how it works.
The input differential pair implements one of the few forms of distortion
cancellation that can be relied upon to work reliably without adjustment –
this is because the transconductance of the input pair is determined by the
physics of transistor action rather than matching of ill-defined parameters
such as beta; the logarithmic relation between Ic and Vbe is proverbially
accurate over some eight or nine decades of current variation.
The voltage signal at the Voltage Amplifier Stage (hereafter VAS) transistor
base is typically a couple of millivolts, looking rather like a distorted
triangle wave. Fortunately the voltage here is of little more than academic
interest, as the circuit topology essentially consists of a transconductance
amp (voltage-difference input to current output) driving into a trans-
resistance (current-to-voltage converter) stage. In the first case the
exponential Vbe/Ic law is straightened out by the differential-pair action,
and in the second the global (overall) feedback factor at LF is sufficient to
linearise the VAS, while at HF shunt Negative Feedback (hereafter NFB)
through Cdom conveniently takes over VAS-linearisation while the overall
feedback factor is falling.
The behaviour of Miller dominant-pole compensation in this stage is
actually exceedingly elegant, and not at all a case of finding the most
60
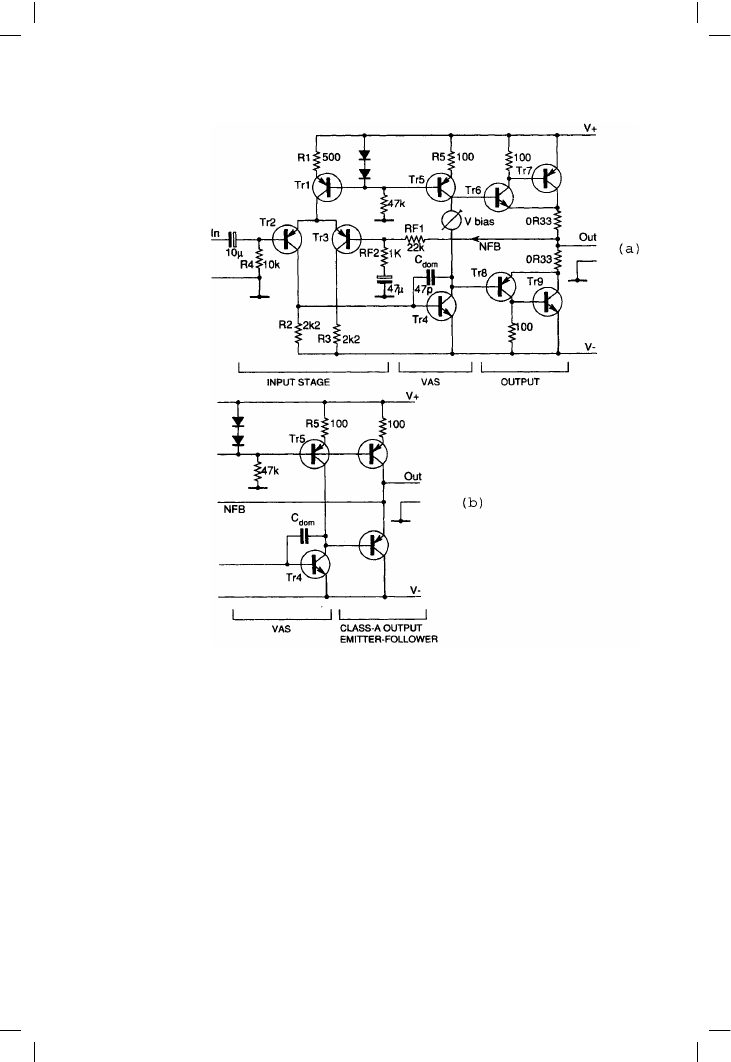
The general principles of power amplifiers
vulnerable transistor and slugging it. As frequency rises and Cdom begins to
take effect, negative feedback is no longer applied globally around the
whole amplifier, which would include the higher poles, but instead is
seamlessly transferred to a purely local role in linearising the VAS. Since
this stage effectively contains a single gain transistor, any amount of NFB
can be applied to it without stability problems.
The amplifier operates in two regions; the LF, where open-loop (o/I) gain is
substantially constant, and HF, above the dominant-pole breakpoint, where
the gain is decreasing steadily at 6 dB/octave. Assuming the output stage is
unity-gain, three simple relationships define the gain in these two regions:
LF gain = g
m
× beta × R
c
Equation 3.1
At least one of the factors that set this (beta) is not well-controlled and so
the LF gain of the amplifier is to a certain extent a matter of pot-luck;
61
Figure 3.1
a A conventional
Class-B power amp
circuit. b With small-
signal Class-A output
emitter-follower
replacing Class-B
output to make a
model amplifier
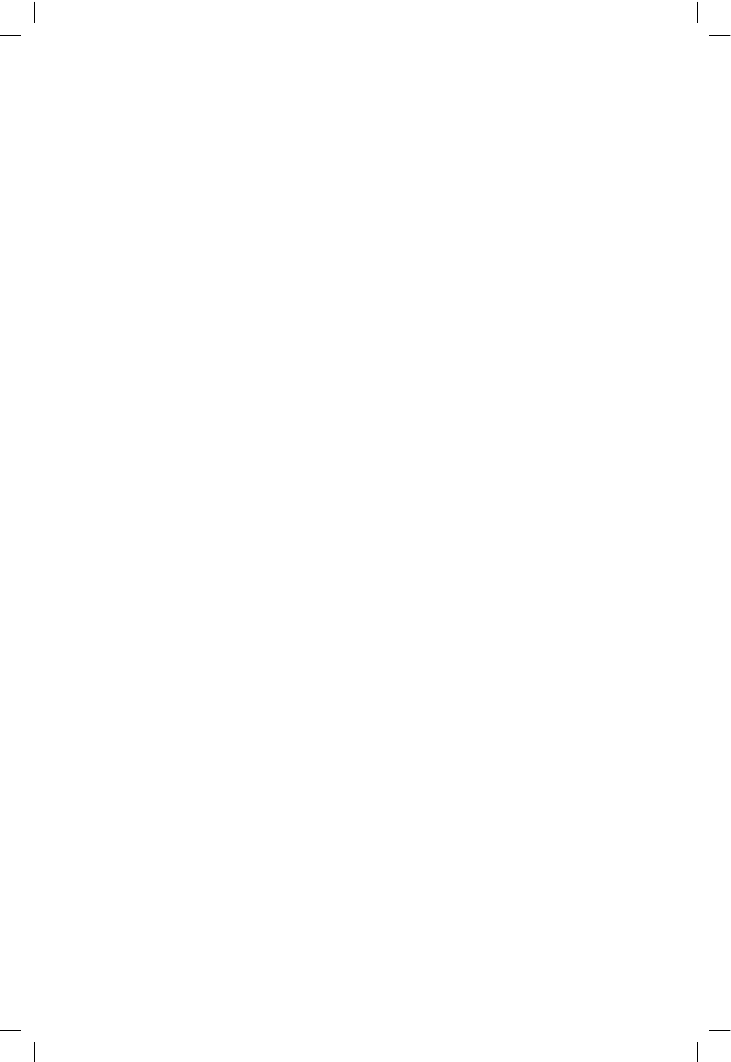
Audio Power Amplifier Design Handbook
fortunately this doesn’t matter, so long as it is high enough to give a suitable
level of NFB to eliminate LF distortion. The use of the word eliminate is
deliberate, as will be seen later. Usually the LF gain, or HF local feedback-
factor, is made high by increasing the effective value of the VAS collector
impedance Rc, either by the use of current-source collector-load, or by
some form of bootstrapping.
The other important relations are:
HF gain = g
m
/ (w
× C
dom
)
Equation 3.2
Dominant pole freq P1 = 1/(w
× C
dom
× beta × R
c
)
Equation 3.3
(where w = 2
× pi × freq).
In the HF region, things are distinctly more difficult as regards distortion, for
while the VAS is locally linearised, the global feedback-factor available to
linearise the input and output stages is falling steadily at 6 dB/octave. For
the time being we will assume that it is possible to define an HF gain (say
N dB at 20 kHz) which will assure stability with practical loads and
component variations. Note that the HF gain, and therefore both HF
distortion and stability margin, are set by the simple combination of the
input stage transconductance and one capacitor, and most components
have no effect on it at all.
It is often said that the use of a high VAS collector impedance provides a
current drive to the output devices, often with the implication that this
somehow allows the stage to skip quickly and lightly over the dreaded
crossover region. This is a misconception – the collector impedance falls to
a few kilohms at HF, due to increasing local feedback through Cdom, and
in any case it is very doubtful if true current drive would be a good thing
– calculation shows that a low-impedance voltage drive minimises
distortion due to beta-unmatched output halves
[1]
, and it certainly
eliminates the effect of Distortion 4, described below.
The advantages of convention
It is probably not an accident that the generic configuration is by a long
way the most popular, though in the uncertain world of audio technology
it is unwise to be too dogmatic about this sort of thing. The generic
configuration has several advantages over other approaches:
!
The input pair not only provides the simplest way of making a DC-
coupled amplifier with a dependably small output offset voltage, but can
also (given half a chance) completely cancel the 2nd-harmonic
distortion which would be generated by a single-transistor input stage.
One vital condition for this must be met; the pair must be accurately
balanced by choosing the associated components so that the two
collector currents are equal. (The typical component values shown in
Figure 3.1 do not bring about this most desirable state of affairs.)
62
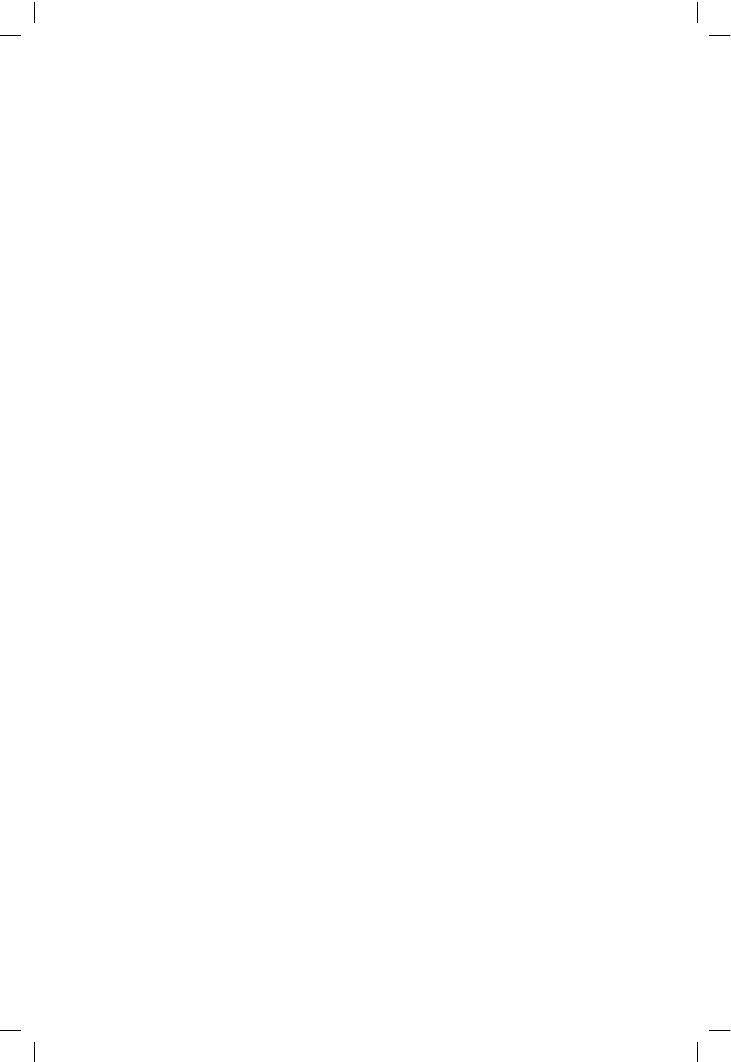
The general principles of power amplifiers
!
The input devices work at a constant and near-equal Vce, giving good
thermal balance.
!
The input pair has virtually no voltage gain so no low-frequency pole
can be generated by Miller effect in the TR2 collector-base capaci-
tance. All the voltage gain is provided by the VAS stage, which makes
for easy compensation. Feedback through Cdom lowers VAS input and
output impedances, minimising the effect of input-stage capacitance,
and the output stage capacitance. This is often known as pole-
splitting
[2]
; the pole of the VAS is moved downwards in frequency to
become the dominant pole, while the input-stage pole is pushed up in
frequency.
!
The VAS Miller compensation capacitance smoothly transfers NFB from
a global loop that may be unstable, to the VAS local loop that cannot be.
It is quite wrong to state that all the benefits of feedback are lost as the
frequency increases above the dominant pole, as the VAS is still being
linearised. This position of Cdom also swamps the rather variable Ccb of
the VAS transistor.
The eight distortions
My original series of articles on amplifier distortion listed seven important
distortion mechanisms, all of which are applicable to any Class-B amplifier,
and do not depend on particular circuit arrangements. As a result of further
experimentation, I have now increased this to eight.
In the typical amplifier THD is often thought to be simply due to the Class-B
nature of the output stage, which is linearised less effectively as the
feedback factor falls with increasing frequency. This is, however, only true
when all the removable sources of distortion have been eliminated. In the
vast majority of amplifiers in production, the true situation is more
complex, as the small-signal stages can generate significant distortion of
their own, in at least two different ways; this distortion can easily exceed
output stage distortion at high frequencies. It is particularly inelegant to
allow this to occur given the freedom of design possible in the small-signal
section.
If the ills that a class-B stage is heir to are included then there are eight
major distortion mechanisms. Note that this assumes that the amplifier is
not overloaded, and has proper global or Nyquist stability and does not
suffer from any parasitic oscillations; the latter, if of high enough frequency,
tend to manifest themselves only as unexpected increases in distortion,
sometimes at very specific power outputs and frequencies.
In Figure 3.2 an attempt has been made to show the distortion situation
diagrammatically, indicating the location of each mechanism within the
amplifier. Distortion 8 is not shown.
63
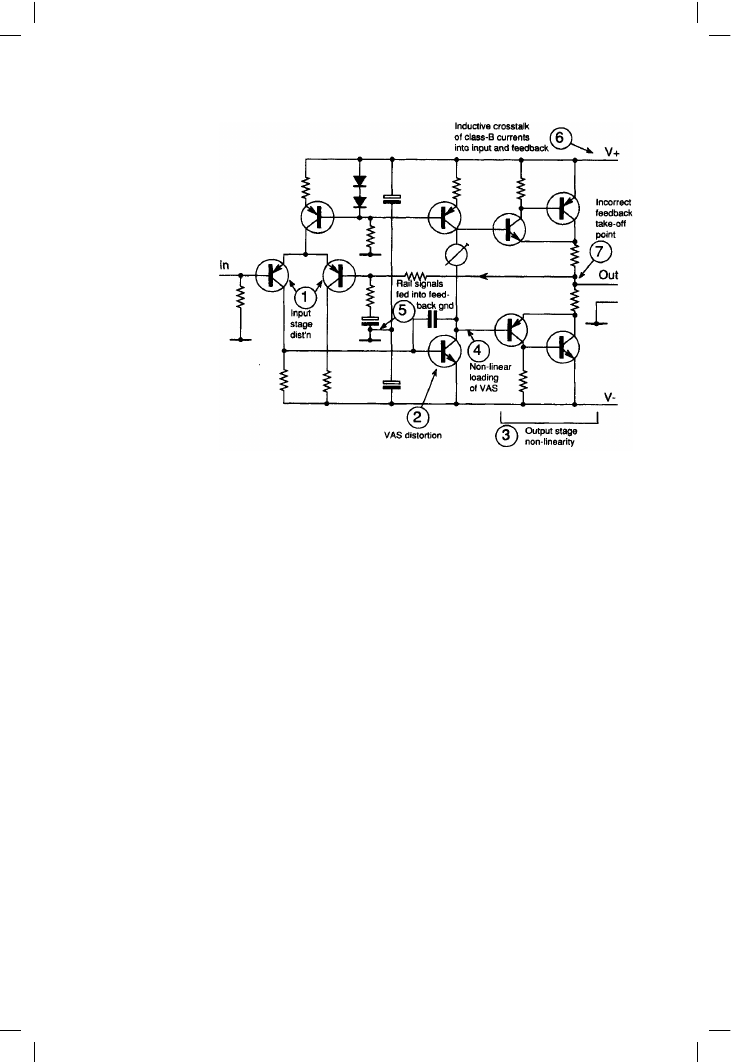
Audio Power Amplifier Design Handbook
Distortion one: input stage distortion
Non-linearity in the input stage. If this is a carefully-balanced differential
pair then the distortion is typically only measurable at HF, rises at 18 dB/
octave, and is almost pure third harmonic. If the input pair is unbalanced
(which from published circuitry it usually is) then the HF distortion emerges
from the noise floor earlier, as frequency increases, and rises at 12 dB/
octave as it is mostly second harmonic.
Distortion two: vas distortion
Non-linearity in the voltage-amplifier stage (which I call the VAS for
concision) surprisingly does not always figure in the total distortion. If it
does, it remains constant until the dominant-pole freq P1 is reached, and
then rises at 6 dB/octave. With the configurations discussed here it is always
second harmonic.
Usually the level is very low due to linearising negative feedback through
the dominant-pole capacitor. Hence if you crank up the local VAS open-
loop gain, for example by cascoding or putting more current-gain in the
local VAS-Cdom loop, and attend to Distortion 4) below, you can usually
ignore VAS distortion.
Distortion three: output stage distortion
Non-linearity in the output stage, which is naturally the obvious source.
This in a Class-B amplifier will be a complex mix of large-signal distortion
and crossover effects, the latter generating a spray of high-order harmonics,
and in general rising at 6 dB/octave as the amount of negative feedback
64
Figure 3.2
The location of the first
seven major distortion
mechanisms. The
eighth (capacitor
distortion) is omitted for
clarity