ВУЗ: Казахская Национальная Академия Искусств им. Т. Жургенова
Категория: Учебное пособие
Дисциплина: Не указана
Добавлен: 03.02.2019
Просмотров: 17401
Скачиваний: 18
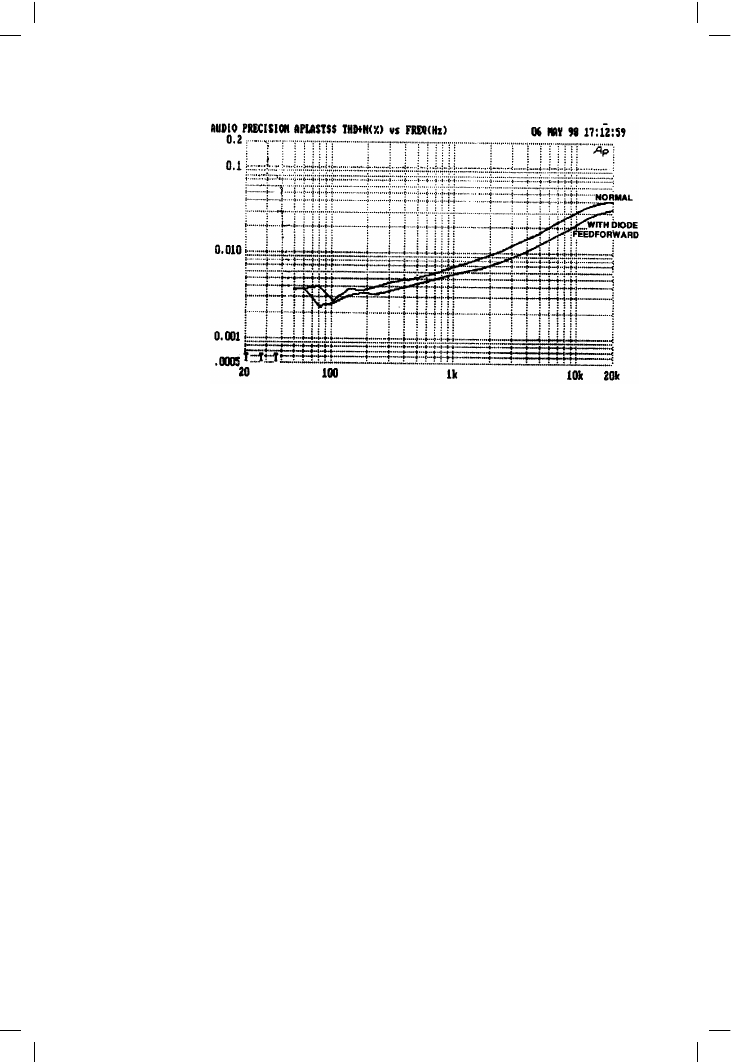
The output stage I
Apart from Load-Invariance, the design also incorporates two new
techniques from the Thermal Dynamics section of this book.
The first technique greatly reduces time lag in the thermal compensation.
With a CFP output stage, the bias generator aims to shadow driver junction
temperature rather than the output junctions. A much faster response to
power dissipation changes is obtained by mounting bias generator
transistor TR8 on top of driver TR14, rather than on the other side of the
heatsink. The driver heatsink mass is largely decoupled from the thermal
compensation system, and the response is speeded up by at least two
orders of magnitude.
The second innovation is a bias generator with an increased temperature
coefficient, to reduce the static errors introduced by thermal losses between
driver and sensor. The bias generator tempco is increased to –4.0 mV/°C. D5
also compensates for the effect of ambient temperature changes.
This design is not described in detail because it closely resembles the
Blameless Class-B amp described on page 176. The low-noise feedback
network is taken from the Trimodal amplifier on page 270; note the
requirement for input bootstrapping if a 10k input impedance is required.
Single-slope VI limiting is incorporated for overload protection, implemented
by TR12, 13. The global NFB factor is once more a modest 30 dB at 20 kHz.
The latest findings
I have recently done further experiments with multiple devices, using three,
four, five and six in parallel. The 2SC2922/2SA1612 complementary pair
were used. In this case the circuit used was somewhat different (see Figure
5.26). With a greater number of devices I was now more concerned about
proper current sharing, and so each device has its own emitter resistor. This
makes it look much more like a conventional paralleled output stage,
135
Figure 5.25
Simple diode
feedforward reduces
distortion with sub-8 !
loads. Measured at
210 W into 2.7 !
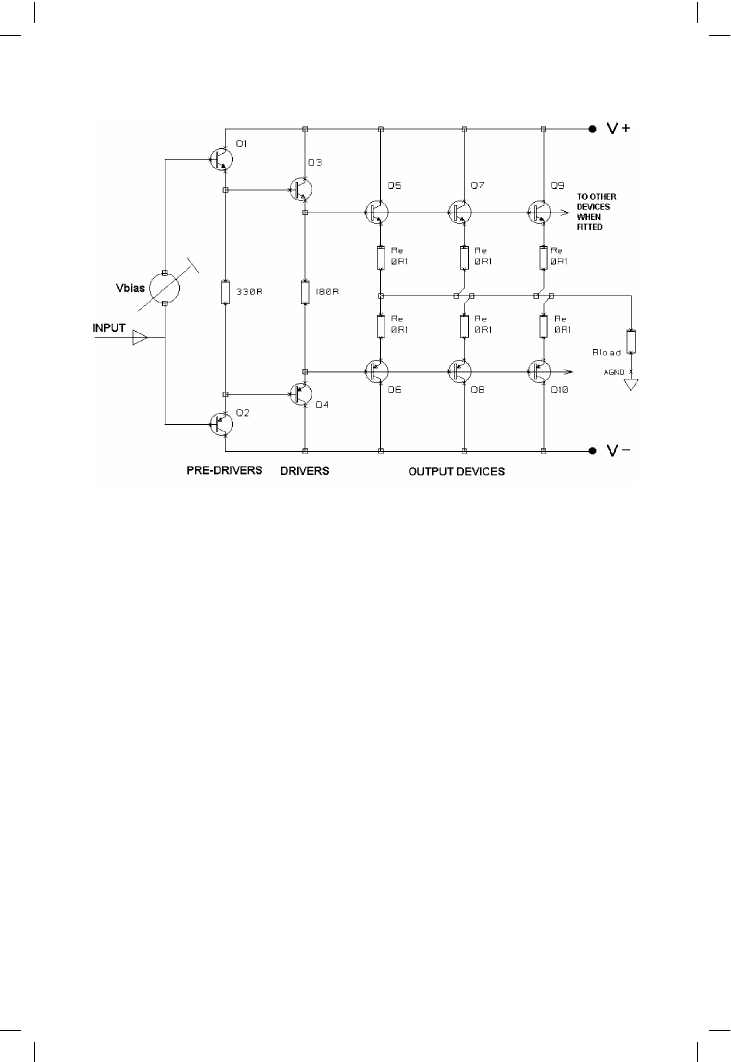
Audio Power Amplifier Design Handbook
which essentially it is. This time I tried both double and the triple-EF output
configurations, as I wished to prove:
(a) that LSN theory worked for both of the common configurations EF and
CFP – it does;
(b) that LSN theory worked for both double and triple versions of the EF
output stage – it does.
For reasons of space only the triple-EF results are discussed here.
Figure 5.27 shows the measured THD results for one complementary pair
of output devices in the triple-EF circuit of Fig 5.25. Distortion is slightly
higher, and the noise floor relatively lower, than in the standard result (Fig
2 in Part 1) because of the higher output power of 50 W/8 !. Figure 5.28
shows the same except there are now two pairs of output devices. Note that
THD has halved at both 8 and 4 ! loads; this is probably due to the larger
currents taken by 8 ! loads at this higher power. Figure 5.29 shows the
result for six devices; 8 ! distortion has almost been abolished, and the 4 !
result is almost as good. It is necessary to go down to a 2 ! load to get the
THD clear of the noise so it can be measured accurately. With six outputs,
driving a substantial amount of power into this load is not a problem.
On a practical note, the more output devices you have, the harder the
amplifier may be to purge of parasitic oscillations in the output stage. This
is presumably due to the extra raw transconductance available, and can be
136
Figure 5.26
The triple-EF output stage used for the measurements described below. ‘Triple’ refers to the fact that there are three
transistors from input to output, rather than the fact that there happen to be three output devices in parallel
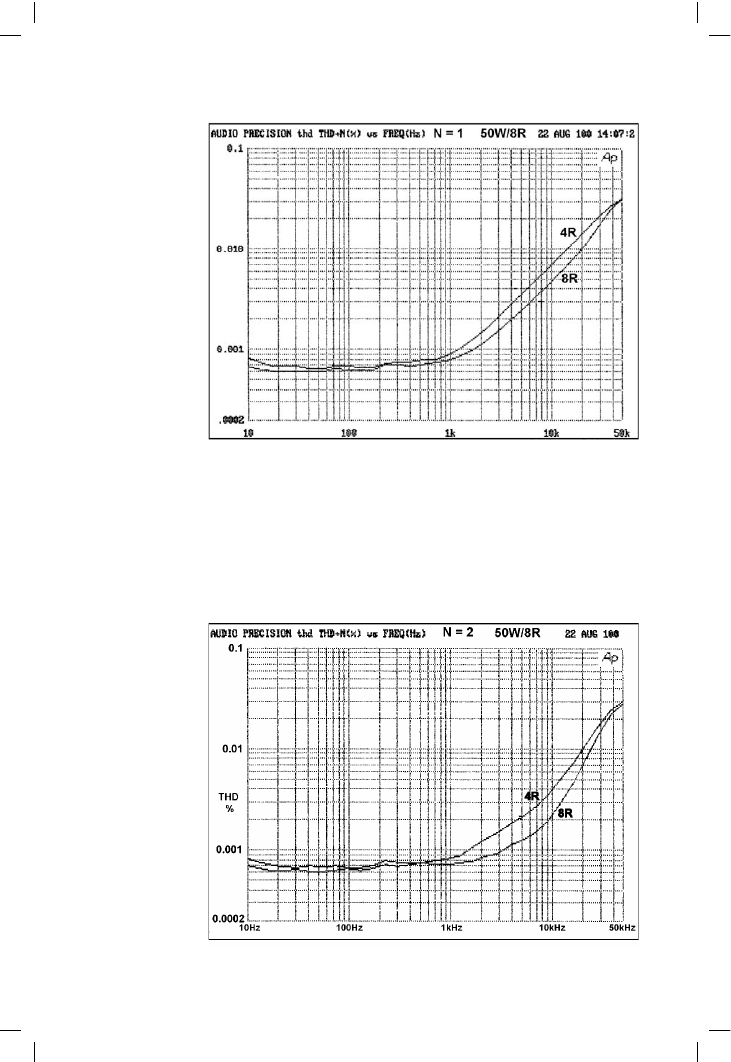
The output stage I
a problem even with the triple-EF circuit, which has no local NFB loops. I
don’t pretend to be able to give a detailed explanation of this effect at the
moment.
Having demonstrated that sustained-beta output devices not only reduce
LSN but also unexpectedly reduce crossover distortion, it seemed worth
checking if using multiple output devices would give a similar reduction at
light loading. I was rather surprised to find they did.
137
Figure 5.27
THD for one pair
(N=1) of output
devices, at 50W/8R
and 100W/4R
Figure 5.28
THD for two pairs
(N=2) of output
devices, at 50W/8R
and 100W/4R. A
definite improvement
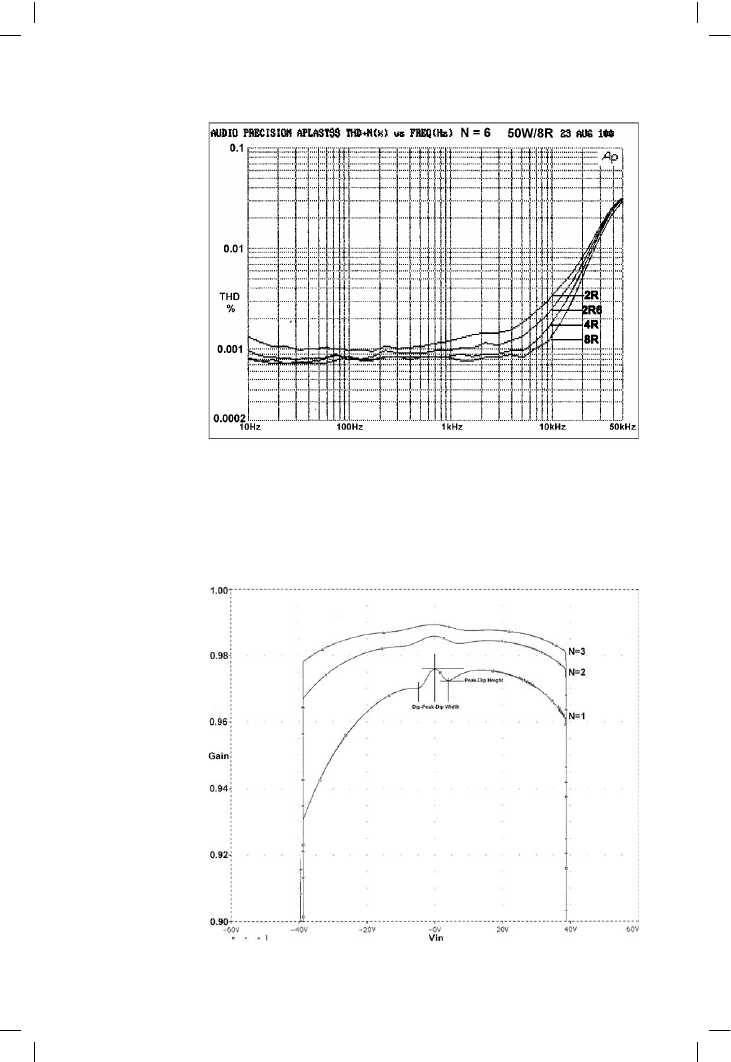
Audio Power Amplifier Design Handbook
Adding more output devices in parallel, while driving an 8-! load, results
in a steady reduction in distortion. Figures 5.27–5.29 show how this works
in reality. The SPICE simulations in Figure 5.30 reveal that increasing the
number N of output devices not only flattens the crossover gain wobble,
but spreads it out over a greater width. This spreading effect is an extra
138
Figure 5.29
THD for six pairs
(N=6) of output
devices, at 50W/8R,
100W/4R,
200W/2R. Note
very low distortion at
8 ohms
Figure 5.30
SPICE simulation of
triple-EF output with
N=1, 2 and 3. As N
increases the
crossover gain
wobble becomes
flatter and more
spread out laterally
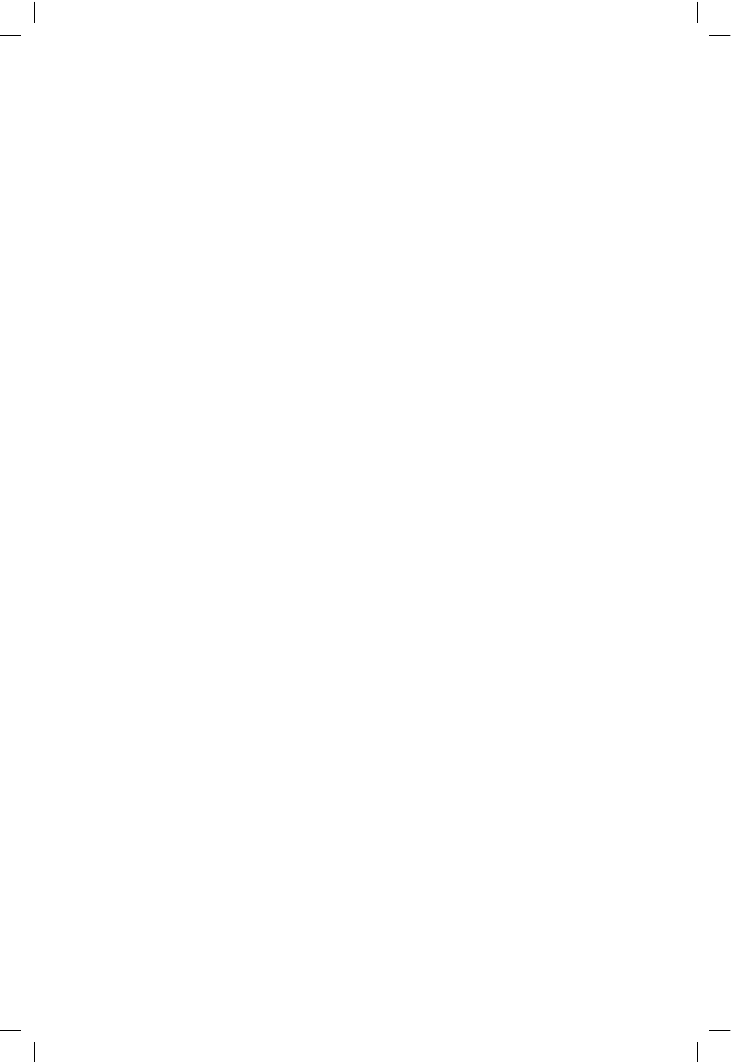
The output stage I
bonus because it means that lower-order harmonics are generated, and at
lower frequencies there will be more negative feedback to linearise them.
(Bear in mind also that a triple-EF output has an inherently wider gain
wobble than the double-EF.) Taking the gain wobble width as the voltage
between the bottoms of the two dips, this appears to be proportional to N.
The amount of gain wobble, as measured from top of the peak to bottom of
the dips, appears to be proportional to 1/N.
This makes sense. We know that crossover distortion increases with heavier
loading, i.e. with greater currents flowing in the output devices, but under
the same voltage conditions. It is therefore not surprising that reducing the
device currents by using multiple devices has the same effect as reducing
loading. If there are two output devices in parallel, each sees half the
current variations, and crossover non-linearity is reduced. The voltage
conditions are the same in each half and so are unchanged. This offers us
the interesting possibility that crossover distortion – which has hitherto
appeared inescapable – can be reduced to an arbitrary level simply by
paralleling enough output transistors. To the best of my knowledge this is a
new insight.
Summary
In conventional amplifiers, reducing the 8 ! load to 4 ! increases the THD
by 2 to 3 times. The figure attained by this amplifier is 1.2 times, and the
ratio could be made even closer to unity by tripling the output devices.
Crossover distortion (Distortion 3b)
In a field like Audio where consensus of any sort is rare, it is a truth
universally acknowledged that crossover distortion is the worst problem
that can afflict Class-B power amplifiers. The problem is the crossover
region, where control of the output voltage must be handed over from one
device to another. Crossover distortion is rightly feared as it generates
unpleasant high-order harmonics, with at least the potential to increase in
percentage as signal level falls.
The pernicious nature of crossover distortion is partly because it occurs
over a small part of the signal swing, and so generates high-order
harmonics. Worse still, this small range over which it does occur is at the
zero-crossing point, so not only is it present at all levels and all but the
lightest loads, but is generally believed to increase as output level falls,
threatening very poor linearity at the modest listening powers that most
people use.
There is a consensus that crossover caused the transistor sound of the
1960s, though to the best of my knowledge this has never actually been
confirmed by the double-blind testing of vintage equipment.
139