ВУЗ: Казахская Национальная Академия Искусств им. Т. Жургенова
Категория: Учебное пособие
Дисциплина: Не указана
Добавлен: 03.02.2019
Просмотров: 17381
Скачиваний: 18
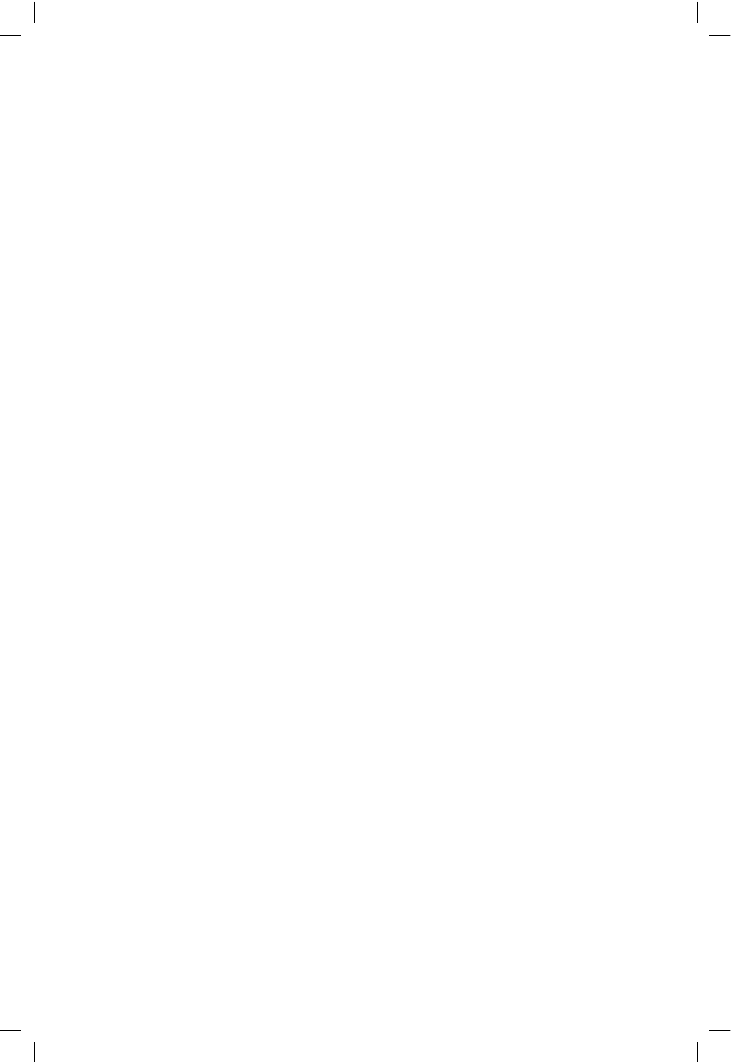
12
Thermal compensation
and thermal dynamics
Why quiescent conditions are critical
In earlier sections of this book we looked closely at the distortion produced
by amplifier output stages, and it emerged that a well-designed Class-B
amplifier with proper precautions taken against the easily-fixed sources of
non-linearity, but using basically conventional circuitry, can produce
startlingly low levels of THD. The distortion that actually is generated is
mainly due to the difficulty of reducing high-order crossover non-linearities
with a global negative-feedback factor that declines with frequency; for 8 !
loads this is the major source of distortion, and unfortunately crossover
distortion is generally regarded as the most pernicious of non-linearities. For
convenience, I have chosen to call such an amplifier, with its small signal
stages freed from unnecessary distortions, but still producing the crossover
distortion inherent in Class-B, a Blameless amplifier (see Chapter 3).
Page 145 suggests that the amount of crossover distortion produced by the
output stage is largely fixed for a given configuration and devices, so the
best we can do is ensure the output stage runs at optimal quiescent
conditions to minimise distortion.
Since it is our only option, it is therefore particularly important to minimise
the output-stage gain irregularities around the crossover point by holding
the quiescent conditions at their optimal value. This conclusion is
reinforced by the finding that for a Blameless amplifier increasing quiescent
current to move into Class-AB makes the distortion worse, not better, as gm-
doubling artefacts are generated. In other words the quiescent setting will
only be correct over a relatively narrow band, and THD measurements
show that too much quiescent current is as bad (or at any rate very little
better) than too little.
325
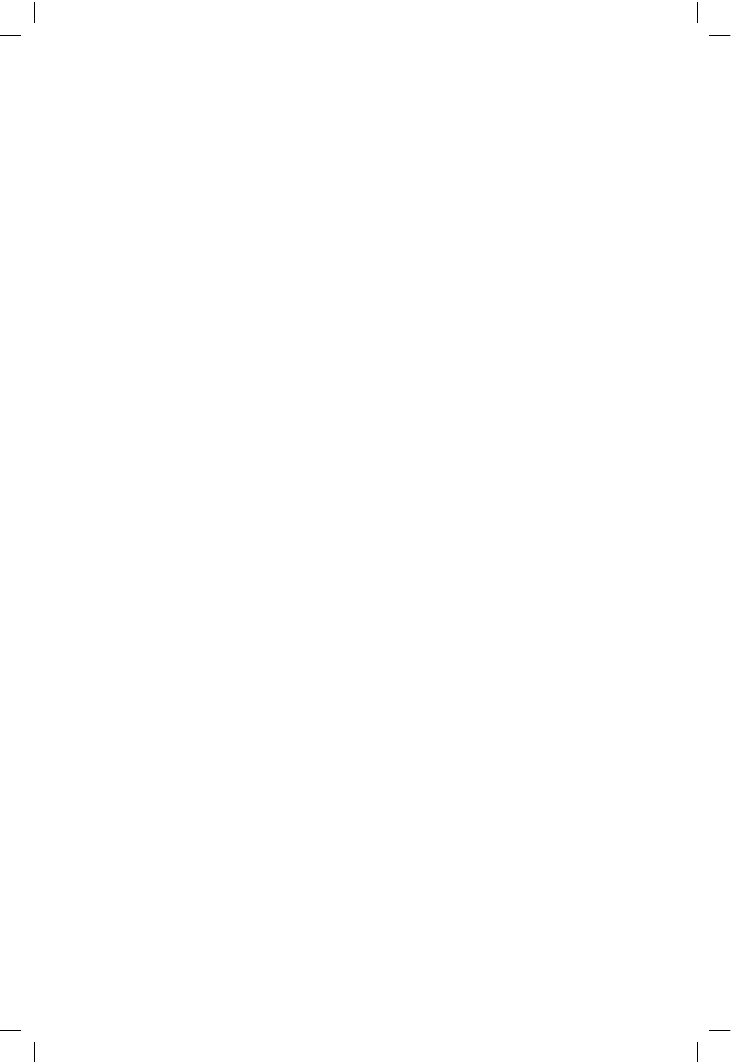
Audio Power Amplifier Design Handbook
The initial quiescent setting is simple, given a THD analyser to get a good
view of the residual distortion; simply increase the bias setting from
minimum until the sharp crossover spikes on the residual merge into the
noise. Advancing the preset further produces edges on the residual that
move apart from the crossover point as bias increases; this is gm-doubling
at work, and is a sign that the bias must be reduced again.
It is easy to attain this optimal setting, but keeping it under varying
operating conditions is a much greater problem because quiescent current
(Iq) depends on the maintenance of an accurate voltage-drop Vq across
emitter resistors Re of tiny value, by means of hot transistors with varying
Vbe drops. It’s surprising it works as well as it does.
Some kinds of amplifier (e.g. Class-A or current-dumping types) manage to
evade the problem altogether, but in general the solution is some form of
thermal compensation, the output-stage bias voltage being set by a
temperature-sensor (usually a Vbe-multiplier transistor) coupled as closely
as possible to the power devices.
There are inherent inaccuracies and thermal lags in this sort of arrange-
ment, leading to program-dependency of Iq. A sudden period of high
power dissipation will begin with the Iq increasing above the optimum, as
the junctions will heat up very quickly. Eventually the thermal mass of the
heatsink will respond, and the bias voltage will be reduced. When the
power dissipation falls again, the bias voltage will now be too low to match
the cooling junctions and the amplifier will be under-biased, producing
crossover spikes that may persist for some minutes. This is very well
illustrated in an important paper by Sato et al
[1]
.
Accuracy required of thermal compensation
Quiescent stability depends on two main factors. The first is the stability of
the Vbias generator in the face of external perturbations, such as supply
voltage variations. The second and more important is the effect of
temperature changes in the drivers and output devices, and the accuracy
with which Vbias can cancel them out.
Vbias must cancel out temperature-induced changes in the voltage across
the transistor base-emitter junctions, so that Vq remains constant. From the
limited viewpoint of thermal compensation (and given a fixed Re) this is
very much the same as the traditional criterion that the quiescent current
must remain constant, and no relaxation in exactitude of setting is
permissible.
I have reached some conclusions on how accurate the Vbias setting must
be to attain minimal distortion. The two major types of output stage, the EF
and the CFP, are quite different in their behaviour and bias requirements,
and this complicates matters considerably. The results are approximate,
326
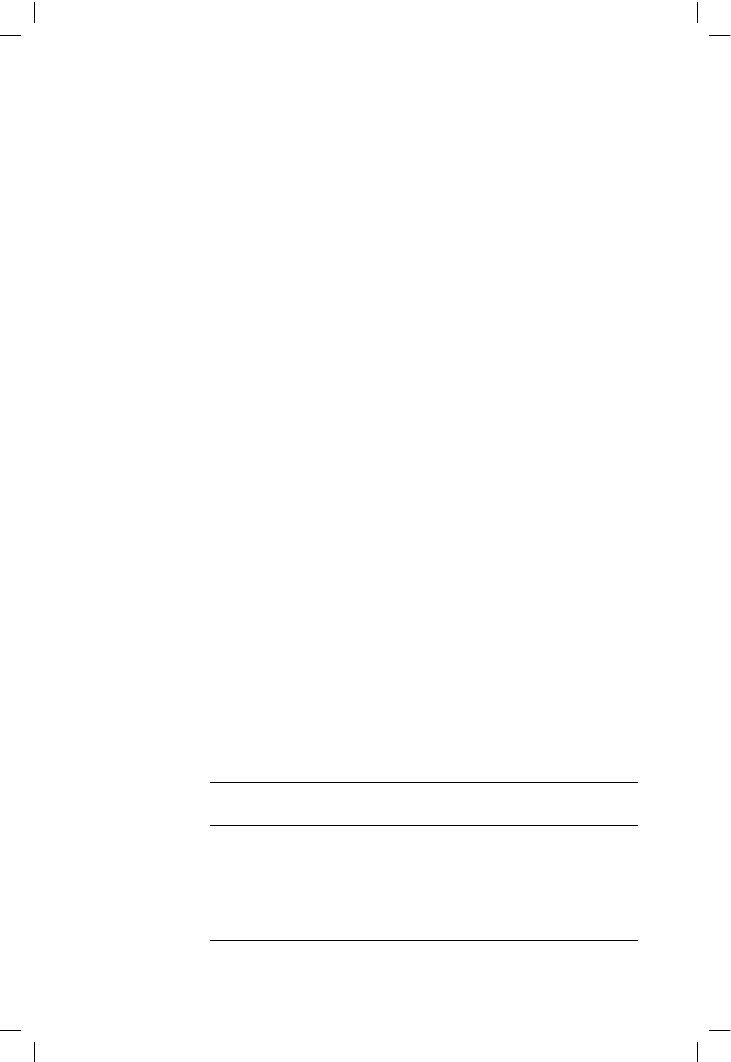
Thermal compensation and thermal dynamics
depending partly on visual assessment of a noisy residual signal, and may
change slightly with transistor type, etc. Nonetheless, Table 12.1 gives a
much-needed starting point for the study of thermal compensation.
From these results, we can take the permissible error band for the EF stage
as about +/–100 mV, and for the CFP as about +/–10 mV. This goes some
way to explaining why the EF stage can give satisfactory quiescent stability
despite its dependence on the Vbe of hot power transistors.
Returning to the PSpice simulator, and taking Re = OR1, a quick check on
how the various transistor junction temperatures affect Vq yields:
!
The EF output stage has a Vq of 42 mV, with a Vq sensitivity of –2 mV/°C
to driver temperature, and –2 mV/°C to output junction temperature. No
surprises here.
!
The CFP stage has a much smaller Vq (3.1 mV) Vq sensitivity is –2 mV/°C
to driver temperature, and only –0.1 mV/°C to output device tem-
perature. This confirms that local NFB in the stage makes Vq relatively
independent of output device temperature, which is just as well as Table
12.1 shows it needs to be about ten times more accurate.
The CFP output devices are about 20 times less sensitive to junction
temperature, but the Vq across Re is something like 10 times less; hence the
actual relationship between output junction temperature and crossover
distortion is not so very different for the two configurations, indicating that
as regards temperature stability the CFP may only be twice as good as the
EF, and not vastly better, which is perhaps the common assumption. In fact,
as will be described, the CFP may show poorer thermal performance in
practice.
In real life, with a continuously varying power output, the situation is
complicated by the different dissipation characteristics of the drivers as
output varies. See Figure 12.1, which shows that the CFP driver dissipation
is more variable with output, but on average runs cooler. For both
configurations driver temperature is equally important, but the EF driver
dissipation does not vary much with output power, though the initial drift
at switch-on is greater as the standing dissipation is higher. This, combined
with the two-times-greater sensitivity to output device temperature and the
327
Table 12.1
Vbias tolerance
for 8 !
EF output
CFP output
Crossover spikes obvious
Underbias
2.25 V
1.242 V
Spikes just visible
Underbias
2.29
1.258
Optimal residual
Optimal
2.38
1.283
gm-doubling just visible
Overbias
2.50
1.291
gm-doubling obvious
Overbias
2.76
1.330
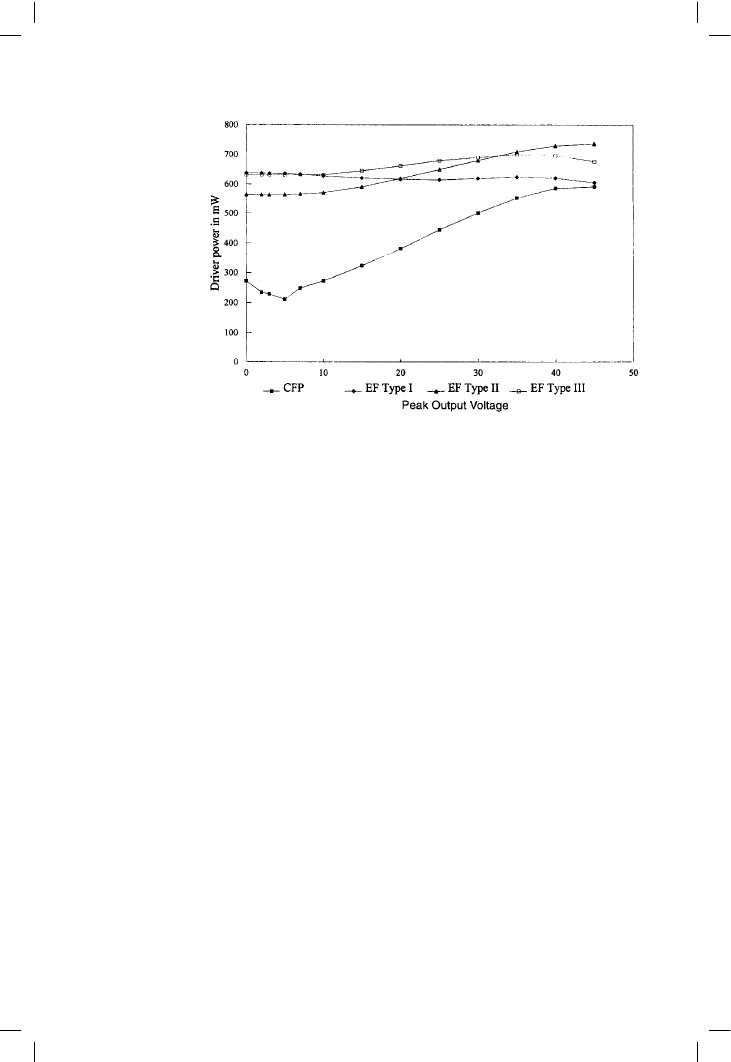
Audio Power Amplifier Design Handbook
greater self-heating of the EF output devices, may be the real reason why
most designers have a general feeling that the EF version has inferior
quiescent stability. The truth as to which type of stage is more thermally
stable is much more complex, and depends on several design choices and
assumptions.
Having assimilated this, we can speculate on the ideal thermal compensa-
tion system for the two output configurations. The EF stage has Vq set by the
subtraction of four dissimilar base-emitter junctions from Vbias, all having
an equal say, and so all four junction temperatures ought to be factored into
the final result. This would certainly be comprehensive, but four
temperature-sensors per channel is perhaps overdoing it. For the CFP stage,
we can ignore the output device temperatures and only sense the drivers,
which simplifies things and works well in practice.
If we can assume that the drivers and outputs come in complementary pairs
with similar Vbe behaviour, then symmetry prevails and we need only
consider one half of the output stage, so long as Vbias is halved to suit. This
assumes the audio signal is symmetrical over timescales of seconds to
minutes, so that equal dissipations and temperature rises occur in the top
and bottom halves of the output stage. This seems a pretty safe bet, but the
unaccompanied human voice has positive and negative peak values that
may differ by up to 8 dB, so prolonged acapella performances have at least
the potential to mislead any compensator that assumes symmetry. One
amplifier that does use separate sensors for the upper and lower output
sections is the Adcom GFA-565.
For the EF configuration, both drivers and outputs have an equal influence
on the quiescent Vq, but the output devices normally get much hotter than
328
Figure 12.1
Driver dissipation
versus output level. In
all variations on the
EF configuration,
power dissipation
varies little with
output; CFP driver
power however varies
by a factor of two or
more
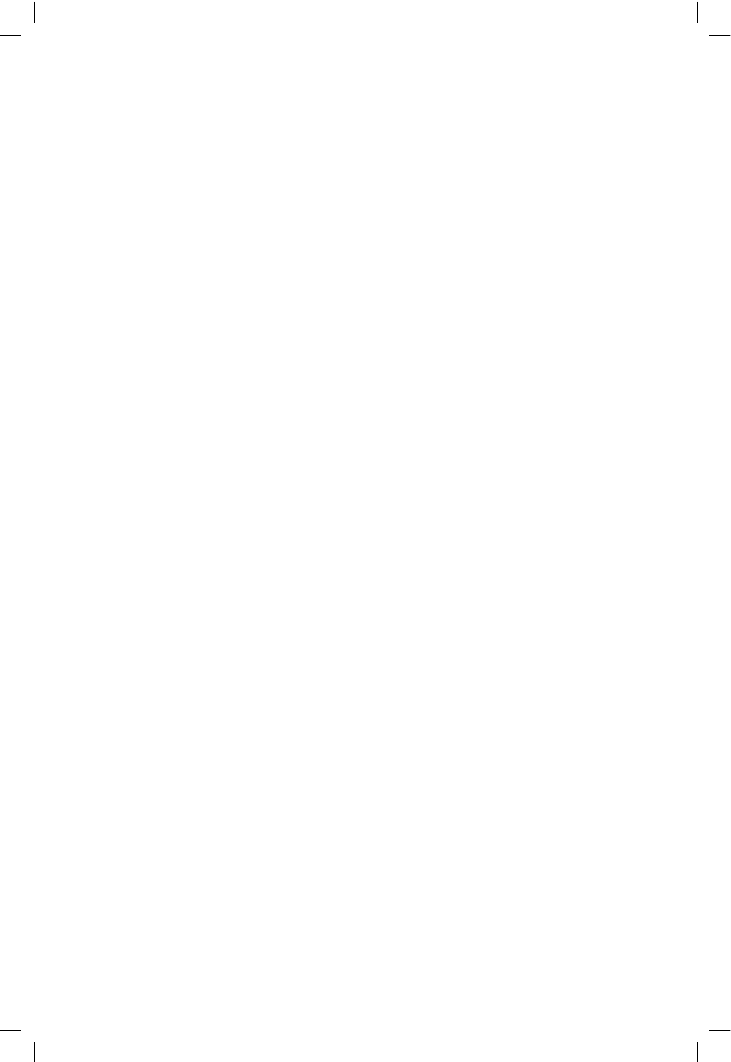
Thermal compensation and thermal dynamics
the drivers, and their dissipation varies much more with output level. In this
case the sensor goes on or near one of the output devices, thermally close
to the output junction. It has been shown experimentally that the top of the
TO3 can is the best place to put it, see page 335. Recent experiments have
confirmed that this holds true also for the TO3P package, (a large flat plastic
package like an overgrown TO220, and nothing like TO3) which can easily
get 20 degrees hotter on its upper plastic surface than does the underlying
heatsink.
In the CFP the drivers have most effect and the output devices, although still
hot, have only one-twentieth the influence. Driver dissipation is also much
more variable, so now the correct place to put the thermal sensor is as near
to the driver junction as you can get it.
Schemes for the direct servo control of quiescent current have been
mooted
[2]
, but all suffer from the difficulty that the quantity we wish to
control is not directly available for measurement, as except in the complete
absence of signal it is swamped by Class-B output currents. In contrast the
quiescent current of a Class-A amplifier is easily measured, allowing very
precise feedback control; ironically its value is not critical for distortion
performance.
So: just how accurately must quiescent current be held? This is not easy to
answer, not least because it is the wrong question. Page 151 established
that the crucial parameter is not quiescent current (hereafter Iq) as such, but
rather the quiescent voltage-drop Vq across the two emitter resistors Re.
This takes a little swallowing – after all people have been worrying about
quiescent current for 30 years or more – but it is actually good news, as the
value of Re does not complicate the picture. The voltage across the output
stage inputs (Vbias) is no less critical, for once Re is chosen Vq and Iq vary
proportionally. The two main types of output stage, the Emitter-Follower
(EF) and the Complementary Feedback Pair (CFP) are shown in Figure 12.2.
Their Vq tolerances are quite different.
From the measurements on page 327 above the permissible error band for
Vq in the EF stage is +/–100 mV, and for the CFP is +/–10 mV. These
tolerances are not defined for all time; I only claim that they are realistic
and reasonable. In terms of total Vbias, the EF needs 2.93 V +/–100 mV, and
the CFP 1.30 V +/–10 mV. Vbias must be higher in the EF as four Vbe’s are
subtracted from it to get Vq, while in the CFP only two driver Vbe’s are
subtracted.
The CFP stage appears to be more demanding of Vbias compensation than
EF, needing 1% rather than 3.5% accuracy, but things are not so simple. Vq
stability in the EF stage depends primarily on the hot output devices, as EF
driver dissipation varies only slightly with power output. Vq in the CFP
depends almost entirely on driver junction temperature, as the effect of
output device temperature is reduced by the local negative-feedback;
329